What's going on?
(brief overview of dust cloud model)
Brief
History of this amateur observing project
WD1145
basic info
Waterfall
Overview
Details of gas
disk model
Orbit
diagrams
K2
Simulations
Traditional
abstract and introduction
Summary
of results (activity level vs. date, stacked
light curves, waterfall plots, SED)
The Poet Within Me
Finder image
References
Related
links (including a link to a very
large web pages with many detailed light curves)
What's
Going On? - A Layman's Overview
"...WD1145 is showing us what our solar system
will be like for most of its existence!"
The WD1145 system consists of a typical size
white dwarf (WD) that is orbited by several
asteroid-size objects (possibly leftover planets).
Dust is being produced by fragments (that have broken
away from the asteroids) by one of several possible
mechanisms. Some dust evaporates to its constituent
atoms (sublimation), and they end up in an inner gas
disk very close to the WD, which then ends up in the
WD's atmosphere (causing the "metal" absorption
lines). The dust that doesn't evaporate forms a
"debris disk" that blocks star light. This disk will
have the appearance of a ring system. We think it
extends from the A-asteroid orbit, at 94 WD radii, to
at least 110 radii, and maybe as far as 150 radii. Our
simplest model interpretation has the several asteroid
orbits and debris disk in the same orbit plane,
inclined 89.5 degrees (i.e., tilted 0.5 degrees for
edge-on). Here's a diagram illustrating that:
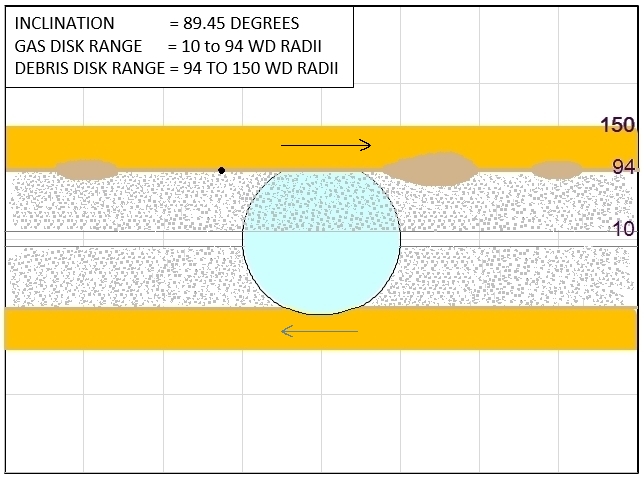
An
artistic representation
of how WD1145
would look from
the Earth. The
black dot
represents the
A-asteroid,
moving to the
right and
about to
produce a
transit of
0.08% (too
small for
detection).
The three
brown ovals
are dust
clouds
produced by
fragments that
have broken
off the
A-asteroid.
Our
light curves
show fades
(also called
"dips")
produced by
dust clouds
that expand
and cover part
of the WD
disk. The
middle dust
cloud in this
depiction is
large enough
to have produced
a 4%
dip (which is
detectable
using amateur
telescopes).
The
dark tan bands
are a debris
disk, or ring
system,
consisting of
spread-out
dust
clouds. The
inner debris
disk is
opaque, and
may become
transparent in
the outer
region (due
to low dust
density).
Small dust
particles that
migrate
inward, toward
the WD, and
become so hot
that the
constituent
atoms
sublimate and
never
re-condense,
form a disk of
metal atoms
(such as iron,
calcium,
aluminum,
etc);
it is referred
to the inner
gas disk.
The metal
atoms absorb
light at only
specific
wavelengths,
so most of the
WD's light
passes through
the gas disk,
and it is therefore
essentially
transparent.
We believe
that both
disks have as
their source
dust from
fragments that
have broken
off the
A-asteroid
(and 5 others,
in slightly
larger orbits
in the debris
disk). The
numbers on the
right edge
show radial
distance of
edges of the
ring system:
the inner gas
ring begins at
10 x WD
radius, the
A-asteroid and
associated
fragments are
at 94 x WD
radius, the
inner edge of
the debris
disk is also
at 94 x WD
radius, and
the outer edge
of debris disk
at ~ 150 x WD
radius.
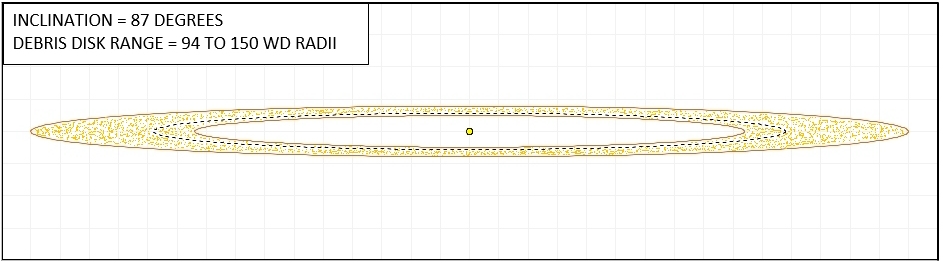
View of larger area for the purpose of showing the
entire debris disk (gas disk not shown), using an
exaggerated tilt of 87 degrees. The dashed line
within the disk corresponds to an asteroid in the
F-orbit, identified by Vanderburg et al (2015) with
an orbit period of 4.858 hrs. For both panels the
size of the WD star is "to scale." WD1145's
debris disk "ring system" has to be "optically
thick" in order to account for measurements of a
"near IR excess" (i.e., measurements at 3.4 and 4.6
micron wavelength by the WISE spacecraft).
Asteroids and comets are thought to be in orbits
that are shrinking (for reasons not yet established).
As an asteroid migrates inward toward the WD the
surface surrounding the asteroid defined by "equal
gravity and centrifugal forces" also shrinks. An
object located just outside this surface (also called
a "Roche lobe boundary" and a "Hill sphere boundary")
would drift away from the asteroid and begin to orbit
the WD. When an asteroid has moved so close to the WD
that the "Hill sphere boundary" reaches the asteroid
end facing the WD, loose material (fragments) at that
location are free to simply drift away from the
asteroid and begin to orbit the WD. The fragment's
orbit will be slightly smaller than the asteroid's
orbit (closer to the white dwarf by ~ 4 times the long
radius of the asteroid).
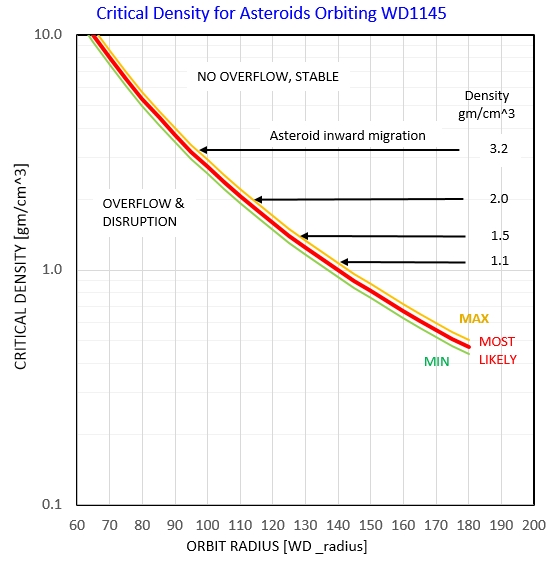
Inward migration track of 4 asteroids toward the
white dwarf until the asteroid's Roche lobe boundary
shrinks to the size of the asteroid, causing
"overflow" at the end of the asteroid facing the
white dwarf. The lowest density object (1.1 gm/cm2,
a comet), begins an overflow when it reaches a
distance of ~ 140 times the white dwarf radius. The
densest asteroid (3.2 gm/cm3) begins an
overflow when it reaches a distance of ~ 94 times
the white dwarf radius. The overflow from all of
these object produces the debris disk, extending
from ~ 94 to 140 times the white dwarf radius. (The
3 curves correspond to uncertainties of the white
dwarf's size and mass, as well as the uncertainty of
the football-shaped object's volume when the Roche
lobe surface shrinks to the size of the asteroid;
adopted volume ratio = 52 ± 4 %.)
Assuming
the A-asteroid has an elongated shape (like
a football) the asteroid's size is estimated
to be 540 ± 50 km (in long direction). This
is based on the difference in orbital period
between the A-asteroid and the fragments
that have broken away and produce the dust
clouds that transit the white dwarf. To
understand why the dust-producing fragments
are in slightly smaller orbits than the
asteroid that they broke away from, it is
necessary to refer to the "Hill sphere
model" proposed by Dr. Saul Rappaport. In
this model the asteroid orbits so close to
the white dwarf star that the strength of
the gravitational fields of the asteroid and
star (adjusting
for centrifugal force) are
the same at the part of the asteroid's
surface facing the star. Any loose fragment
of the asteroid at this "L1 point" on the
asteroid surface can just float away and
orbit the star. The orbit will be at a
distance from the star that is slightly
smaller than the distance of the fragment
when it floated away (the
"slightly smaller" part is due
to a gravity pull on the
fragment as it floats away).
The difference in average orbit distance
(asteroid "mother-ship" versus fragment)
will be ~ 4 times the size of the asteroid
(radial distance from asteroid center to
surface facing the white dwarf). Since
orbital period is dictated by orbit size, we
can use the difference in orbit period of
the fragments with respect to the asteroid
to determine the size of the asteroid. If
this model is correct then the A-asteroid
diameter (longest dimension of a
football-shape) is ~ 60%
that for Ceres,
and the mass of the A-asteroid is ~ 1/10 of
Ceres (taking into account that the volume
of a football shape is ~ 50% of the volume
of a sphere with radius of the longest
dimension). This "Hill sphere model" is
still just a suggestion, awaiting
confirmation.
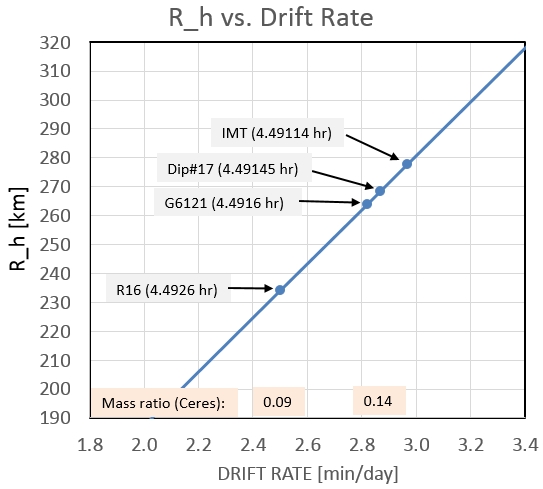
Relationship between fade feature drift
rate with respect to the A-asteroid
ephemeris and A-asteroid size. A consensus
for measured drift rate is 2.85
minutes/day, corresponding to a radius of
~ 270 km (longest dimension of a
football-shaped asteroid). The asteroid
mass is estimated to be ~ 1/10th that of
our solar system's asteroid Ceres.
The manner in which dust clouds migrate
outward (by small distances) to form a
debris disk of dust, and inward toward the
WD to form a gas disk, are the subject of
continued speculation and modeling analysis.
The inward migration involves the breakup of
dust particles to constituent atoms, which
form an inner "circumstellar" disk of atoms
(extending from 10 to 64 WD radii, as
suggested by Redfield et al., 2016). This
inner disk is a gas, not dust. Gas closer
than some distance (such as 10 WD radii) may
be captured by the star's magnetosphere and
quickly brought to the star's surface (for
more on this model, see link).
The outer debris disk (pictured above, from
94 to ~ 150 WD radii) consists of dust
particles (maybe some gas). Both the inner
gas disk and the outer debris disk change
slowly, whereas their source, the dust
clouds at 94 WD radii (producing the
light curve fade events that we observed
during the past 8 months),
can change abruptly due to either collisions
between fragments or breakup without
collision involvement.
Since dust clouds should disperse on
timescales of a few days (due to orbit
shearing) there must be a component of
continual dust production. The mechanism for
dust production is also the subject of much
speculation, and will be the subject of
future publications by modelers. I'm not a
modeler, but I have suggested the following
mechanisms:
1) Continual micro-meteoroid
bombardment of the fragments by previously
released and dispersed dust clouds.
2) Heating of fragment surfaces
that are favorably oriented (facing the WD
star for part of each orbit), causing
surface temperature to rise to 1700 K (or ~
1850 K within craterlet bottoms), causing
melting of minerals.
3) Spinning of fragments that
is fast enough for centrifugal forces to
move molten material to the edges where it
is thrown off to form a dust cloud.
4) Spinning of fragments that
consist of rubble piles held together
initially by inter-molecular forces, whose
loss of cohesion is continual, leading to a
continual ejection of dust with a size
distribution of large range. The smallest
particles should be pushed away from the WD
by radiation pressure, causing them to be in
larger orbits with longer periods, and this
should produce a spreading of dip structure
to larger phases (this spreading pattern is
supported by the observations).
In addition to some mechanism for continual
dust production, collisions (or nearby
passes) of fragments with other fragments
should produce sudden increases of dust
cloud density and size. There may be
thousands of fragments, and it's only after
a collision (or near pass) between them that
they "come alive" for a few days, weeks or
months. The most interesting collision
possibility is of a fragment with the
asteroid! The dramatic increase in fade
activity that occurred about a year ago
(sometime between 2015 June and October)
must have been produced by such a
fragment/asteroid collision. Consider the
following record of overall fade activity:
Activity level vs. date. Activity level is
defined as the sum of "area under the curve" for all
dips occurring during a 4.5-hour orbit. A 45-fold
increase is apparent during parts of the 8 months of
our amateur team observations compared with the
Kepler K2 observations 2 years earlier, and it may
have occurred after a hypothetical collision between
a fragment and the "mother ship" asteroid in 2015
September (Event #1). Activity level declined
steadily starting in 2016 April, and by the end of
the first observing season (2015/16) activity had
returned to a level almost as low as before the
September 2015 event. However, the first few
observations of the new observing season (starting
2016 Oct) shows an uptick of activity, with a 2-step
pattern (Events #5 and 6), reaching ~ 75 times the
K2 level of activity (as of 2016 Dec 15). If the
rate of increase continues according to the fitted
model (4 %/day) the star could be totally obscured,
and invisible, sometime in 2017 February. A more
likely limit to activity is 60% "equivalent width",
given that individual dips have never exceeded this
value, and this level of activity is projected to
occur in 2017 January.
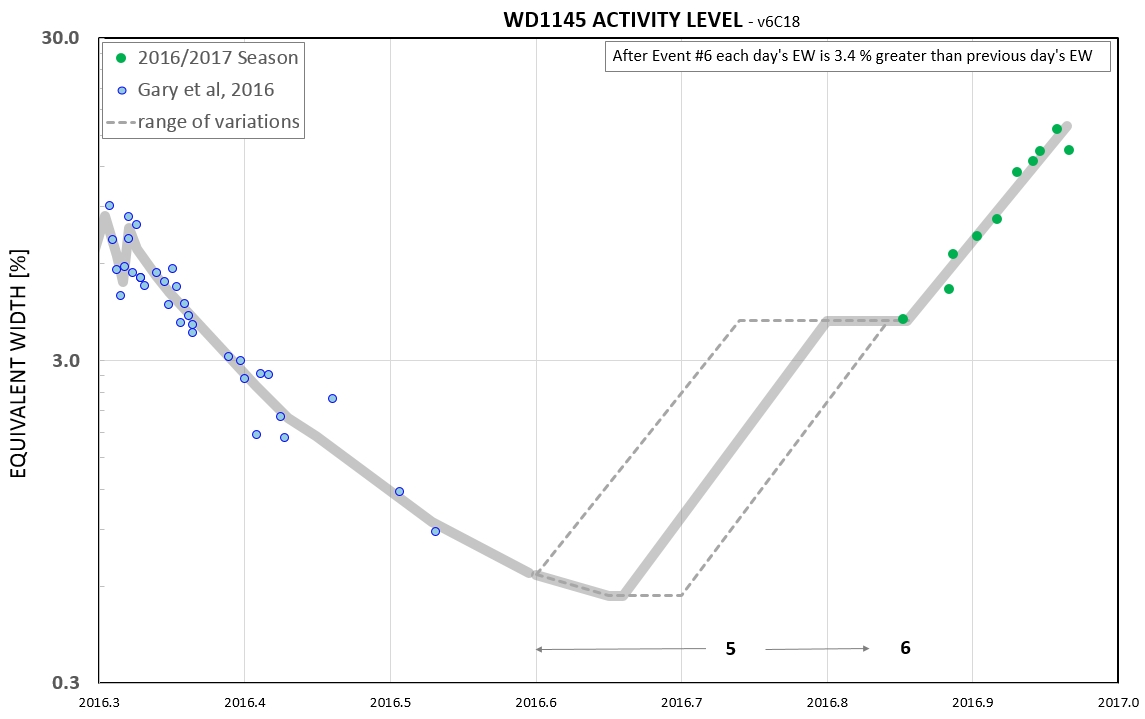
This detail of the gap between observing seasons is
fitted with a two-step rise pattern, suggested by
details of dip groups in light curves from 2016
November and December. The date for the first rise
(Evvent #5) is uncertain, but its shown here to be
late August. The second rise (Event #6, starting
2016 Nov 19) exhibits a uniform rate of increase of
~ 4% per day that has persisted for 5 weeks so far.
If only the pro's had asked for our help
1.5 years ago! We could have provided high density
observations during 2015, like we did one observing
season later. Apparently they didn't think we had the
capability! Now they know, so maybe they'll welcome
our help next year.
Some "new physics" is being stimulated by this star
system. WD1145 may become the "Rosetta Stone" for
understanding the relationship between white dwarfs
and their left-over solar system. Here are some things
about WD1145 that remain to be figured out: 1) How did
planetesimals migrate to smaller orbits, 2) does the
Hill sphere for the closest planetesimal play a role
in forcing mass loss (fragment breakaways), 3) what
mechanisms are responsible for quasi-continuous dust
production (many possibilities), 4) what's the
location and dimension of the debris disk, 5) is any
of the debris disk optically thick, or is all of it
optically thin, 6) was the 2015/16 25-fold rise in
activity caused by a fragment colliding with the
A-asteroid, 7) what is the mechanism for inward
movement of some dust (larger size dust?) for infall
to the WD atmosphere, 8) what is the mechanism for
outward motion (if it exists) for populating the
debris disk, 9) what's the explanation for the large
(250 km/s) range of velocities in the line-of-sight
for spectroscopic metal absorption lines, 10) what's
the size distribution of dust particles (vs location)
in the debris disk (are they all ~ 2 micron in
radius?), 11) do the dust clouds never cause more than
60% loss of WD light because opaque region never
covers > 60% of WD disk, or is it due to dust
covering all of WD disk but has optical thickness of
only ~ 0.8 tau, 12) what fraction of WDs are like
WD1145 in having asteroids undergoing disintegration
(we think it's the same as the 25% to 50% that have
metal absorption line "pollution"), 13) what's the
fraction of time that this WD (and those like it) are
in the heightened activity state (due to collisions of
the asteroid with one of its fragments), etc.
Here's an example of two amateur observers obtaining
the same, identical light curve, illustrating the
feasibility of a 14" telescope for this observing
task:
Light curves spanning a complete dust cloud orbit
of 4.5 hours, that show the same 13 dip features.
The noise levels are the same because the larger
telescope used shorter exposures (15-sec v.s
60-sec).
The light curve, above, illustrates that during high
activity WD1145 is undergoing dips fades most of the
time; the fade level is "out-of-transit" for only 0.5
hour out of a 4.5 hour orbit.
Here's a sampling of light curves, one day apart,
showing normalized flux vs. orbit phase (using the
A-fragment ephemeris for computing phase):
Day-to-day similarity, and slow evolution, of
the fade features are apparent in this series of
light curves spanning a 5-day interval in 2016
April. Phase is defined using an ephemeris that
keeps fragments at the same phase. The group of fade
features near zero phase first appeared on
approximately 2016 Jan 21 (hence the name G6121);
they change slightly on a daily basis. The group of
fade features on the right first appeared on 2016
Apr 20 (hence their name G6420); they are shifting
slowly to the right as they also change shape. The
new feature called "B-dip" first appeared on 2016
Apr 25 (3rd light curve from the bottom), and it
drifted fast to the right each day. It is associated
with an asteroid in a larger orbit than the other
fade features. (The first 5 light curves were made
by professional observer Roi Alonso, using a 32"
telescope on the Canary Islands, IAC80. The last
light curve was made by amateur observer Josch
Hambsch, using his remotely operated 20" telescope
in Chile and controlled from Belgium.)
Here's a sampling of light curves, one per month
(using the same ephemeris for computing phase):
Monthly sample of phased light curves using the
“A-fragment ephemeris.” All observations shown were
made by amateurs using backyard observatories (six
were made with my 14” Meade telescope, one is a
combination of data from my 14” and a 16” telescope,
and two are combined data from 14” and 32”
telescopes). Notice that from month-to-month the
light curves change so much that there's almost no
resemblance; no individual fade retains it's phase
location on month timescales.
The first light curve of the 2015/16 observing
season was made November 01, 2015. By mid-November it
was apparent that dip activity was much higher than
expected, based on Kepler K2 data, and follow-up
ground-based observations conducted by professional
astronomers up to June, 2015. Starting in January most
dips are found at the same phase. Whereas the
day-to-day light curve structures are similar,
allowing identification of the same dip in each light
curve (which enables drift direction and rate to be
measured), on monthly timescales dip structure is
poorly correlated because dust clouds evolve on a
shorter timescales.
Take Home Messages
1) WD1145 has the farthest known asteroid to have
been studied.
2) It is common for white dwarfs to be surrounded by
hundreds, maybe thousands, of fragments that have
broken away from planets and asteroids that are
orbiting too close to the white dwarf. Whereas
most fragments are inactive due to an infrequent
collision rate, when a fragment does collide with
another the pieces will have fresh surfaces for
continuous dust production, causing dust clouds to be
present for days, weeks or months. When a fragment
collides with the "mother ship" asteroid, a dramatic
increase in activity occurs, that may last an entire
year.
3) About 1/3 of all white dwarfs show evidence for
dust falling into their atmospheres. This can be
interpreted in two extreme ways, with the truth
falling somewhere in between: either 1/3 of white
dwarfs spend all their time undergoing dust inflow, or
all white dwarfs spend 1/3 of their time with dust
inflow. Whichever, we are left with the inescapable
finding that it may be common for white dwarfs to
spend either some or all of their entire lifetimes
with planets and asteroids orbiting so close that they
are disintegrating and converting their mass to dust,
some of which makes its way to the white dwarf. Our
sun, like all stars of similar mass, will become a
white dwarf ~ 5 billion years from now, and it will
remain a white dwarf for tens of billions of years.
This is equivalent to stating that most of our solar
system's existence will be spent as a white dwarf that
is pulling planetary bodies inward to orbits where
they will disintegrate. In other words, WD1145 is
showing us what our solar system will be like for most
of its existence!
4) Our sun will become a white dwarf in ~ 5 billion
years, and some of our solar system planets and
asteroids will have orbits that shrink into close
proximity to our white dwarf sun. Our solar system is
destined to "turn to dust" during the ensuing billions
of years, producing intermittent clouds of dust that
block the light of our tiny blue sun, as seen by some
imaginary, far-off observer using telescopes like
those that this amateur observing group has used.
Indeed, it is theoretically possible that the material
of Earth, and the substance of our now living bodies,
will contribute to those fades viewed from afar, in
the distant future.
Do you want a longer, and more detailed explanation of
my view of what's going on with WD1145? Try this PDF link.
Brief
History of this Investigation
About 600 years
ago a white dwarf star, almost as massive as the
sun and slightly larger than the Earth, succeeded
in bringing a planet into an orbit close enough
for the planet to begin to disintegrate. Some of
the dust from that disintegration is ending up in
the star’s atmosphere, which is why we call it a
zombie star. The planet must be in a shrinking
orbit, losing mass as it comes closer to the white
dwarf. It is now smaller than our biggest
asteroid, Ceres, so we refer to it as an asteroid.
We think that fragments of the asteroid are
breaking away and orbiting slightly inside the
asteroid’s orbit, and that they are producing dust
clouds whenever they bump into each other. When
these dust clouds orbit in front of the star they
obscure some of the light and we see a brightness
fade lasting for tens of minutes, typically. The
fade pattern repeats every 4.5 hours, with small
changes, so this must be the orbit period for the
fragment dust clouds. The light of this star is
just now reaching Earth, so as far as we're
concerned these mysterious events are happening
right now - and we're witnessing the behavior of
the farthest away asteroid ever studied.
Kepler K2 data revealed the unusual fades of the
star 2 years ago (Vanderburg et al, 2015). As soon
as it was announced to the public (October, 2015)
I began observing it to see if I could detect
those 1% to 2% Kepler fades. The star is faint,
and very blue, and last November it could only be
observed a couple hours before sunrise. But, yes,
I observed it with my amateur 14” telescope, and I
found those fade events caused by transiting dust
clouds. Lots of transits, and deep ones. This was
unexpected, because professionals had used big
telescopes half a year earlier to see what was
going on, and not much was. But as luck would have
it this star system “came alive” after the pro's,
just in time for me. What a lucky coincidence!
That is what I now refer to as Surprise #1.
The transits I observed were ~ 25 times more
"active" (defined below) than what the pro's
observed. I recruited other amateurs to join in
the fun; Jerry, Tom and Paul joined me in
November. Professional astronomer Saul encouraged
our observing, and by January we had sufficient
new information about the high level of activity
from just our amateur observations that we wrote a
paper and submitted it to an astronomy journal,
MNRAS (now available at arXiv: link).
Saul had the clever idea that the asteroid was so
close to the star that its "Hill sphere" had
shrunk to the size of the asteroid, which "put the
squeeze" on it, leading to mass loss (and a
shrinkage in size as its orbit brought it closer
to the WD star). The Hill sphere can be thought of
approximately as the surface where gravity from
the star is just as strong as from the asteroid.
If the Hill sphere has become the size of the
asteroid, according to our conjecture, a fragment
on the asteroid surface could just float away and
go into orbit around the star. What a wierd solar
system! But for me the most compelling take-home
message is that this solar system is a foretell of
ours, because in 5 billion years our sun will
become a white dwarf, and begin to devour our
solar system of planets using the same physics
that we're now trying to figure out for this white
dwarf, WD1145.
A reviewer of our paper asked why one of our
“waterfall plots” (fade event versus orbit phase,
plotted versus date) showed a pattern of lines
that drifted away from some earlier date. Tom
suggested that this was because the date these
lines converged to was the date of the big
increase in activity. We both viewed that date as
a collision between a fragment and the asteroid.
We still believe that, and the date is sometime in
early September, 2015.
After our paper was done, I intended to relax, and
rest my weary heart that a cardiologist suggested
needed rest. But I had to know what this fickle
star system was doing, because it had a history of
changing every day and producing surprises. So one
beautiful, starry night I sneaked out to my
backyard observatory, unlocked it, and quietly
resumed observing – night after night. I had the
thought that professionals might want to know what
it was doing for their planning of expensive
observations with professional telescopes. I
created a web page with almost daily updates (link).
My Arizona skies were clear almost every night, so
I was producing updates almost daily.
By March I saw that a big change had happened in
mid-January: lots of fragments had broken away
from the asteroid on that date, and coincidentally
there were no more single fragments breaking away.
That was Surprise #2. Because of that surprise I
again recruited more observers to join in the fun:
Tom, Yenal, Paul, Josch and Roi (the only
professional in our group). On April 21 a new big
fade appeared, and a few days later it was
apparent that this was a group of 3 or 4 fragments
that had been created by a collision (between two
fragments) on Apr 20, and the pieces were moving
away from each other. That was Surprise #3, which
supported our idea that backward projection of
drift lines could be used to infer a collision
date (like the more dramatic fragment/asteroid
collision in September). A few days later, on Apr
26, I saw Surprise #4: a new fade appeared that
was deep and only a few minutes in duration (I
later learned that it actually showed up a few
hours earlier in Roi's observations, using a
telescope on the Canary Islands). The amazing
thing about this fade is that it had a
significantly longer orbit period than the others.
In fact, it had a period that was exactly the same
as the Kepler “B period.” The people analyzing
Kepler data found 6 periodicities, which they
named A through F. They weren’t completely
convinced that these 6 periodicities were real,
corresponding to 6 actual asteroids. So Surprise
#4 was confirmation that B was real, which implied
that the other asteroids are also real.
The "observing
season" for this star has ended, so we wrote a
second paper. Saul persuaded me to take the lead
with this one, which made sense because
essentially all the observations of the past 8
months were those that either I made or had
arranged to be made by recruiting others. I also
processed every observer’s data to a format for
display in the same way (a phase-folded light
curve), which made my further analysis easier.
This second WD1145 paper was submitted to MNRAS
(Jul 21) and is currently under review. It is
available as an arXiv posting: link
For an
easy-to-read description of what's going on with
this star system go to the next section ("What's
Going On?").
The
manner in which dust clouds migrate outward
(by small distances) to form a debris disk
of dust, and inward toward the WD to form a
gas disk, are the subject of continued
speculation and modeling analysis. The
inward migration involves the breakup of
dust particles to constituent atoms, which
form an inner "circumstellar" disk of atoms
(extending from 10 to 64 WD radii, as
suggested by Redfield et al., 2016). This
inner disk is a gas, not dust. Gas closer
than some distance (such as 10 WD radii) may
be captured by the star's magnetosphere and
brought to the star's surface. The outer
debris disk (pictured above, from 94 to ~
150 WD radii) consists of dust particles.
Both the inner gas disk and the outer debris
disk change slowly, whereas their source,
dust clouds (at 94 WD radii), can change
abruptly.
Since dust clouds should disperse on
timescales of a few days there must be a
component of continual dust production. The
mechanism for dust production is also the
subject of much speculation, and will be the
subject of future publications by modelers.
I'm not a modeler, but I have suggested the
following mechanisms:
1) Continual
micro-meteoroid bombardment of the fragments
by previously released and dispersed dust
clouds.
2) Heating of fragment
surfaces that are favorably oriented (facing
the WD star for part of each orbit), causing
surface temperature to rise to 1700 K (or ~
1850 K within craterlet bottoms), causing
melting of minerals.
3) Spinning of fragments
that is fast enough for centrifugal forces
to move molten material to the edges where
it is thrown off to form a dust cloud.
In addition to some mechanism for continual
dust production, collisions of fragments
with other fragments should produce sudden
increases of dust cloud density and size.
There may be hundreds, or even thousands of
fragments, and it's only after a collision
between them that they "come alive" for a
few days, weeks or months. The most
interesting collision possibility is of a
fragment with the asteroid! The dramatic
increase in fade activity that occurred
about a year ago (sometime between 2015 June
and October) must have been produced by such
a fragment/asteroid collision.
WD
1145+017 Basic Info
RA/DE =
11:48:33.59 +01:28:59.3 (J2000)
Type: white dwarf, atmosphere mostly Helium
(unionized He absorption lines, only a couple H
lines), metal absorption lines present, Teff
= 15,900 K
V-mag = 17.25, B-V = -0.08, r'-mag = 17.5
IR excess seen in WISE Ch#1 & 2 (3.4 and 4.6
micron wavelength): T_brightness = 1145 K, solid
angle = 116 x WD disk
WD distance from Earth ~ 174 pc (570 lt. yrs)
WD star diameter ~ 1.34 × Earth. WD star mass ~
0.6 × sun. WD star density ~ 4 x 105
[gm/cm3]
WD asteroid A (and its fragments) orbit radius ~
0.00541 a.u. (1.16 × our sun's radius, 94 × WD1145
radius). Apparent size of WD star as viewed from
A-asteroid (& fragments) = 1.26 degrees
(diameter).
A-asteroid orbital period = 4.500 hours, mass ~
0.12 × Ceres mass; diameter ~ 450 to 600 km.
Asteroid orbital velocity ~ 315 km/sec.
A-fragment diameter typically a few km, mass ~ 1021
to 1022 [gram]. Orbital period of
fragments = 4.4912 hours (with some variation)
A-fragment dust cloud size large enough to cover ~
60% of WD star; elongated (mostly in trailing
direction), width perpendicular to orbit plane
comparable to WD star diameter.
A-fragment dust production lifetime = from weeks
to several months. Dust production is of two
types: abrupt (collisions) and quasi-continuous
(many theories).
Dust "loss" is due to "radial shear" due to dust
distribution in different orbits. Dust migration
is inward (to WD star) and outward (to debris
disk) by mechanisms not yet understood.
Tilt of orbits of asteroids A through F (and their
fragments) estimated to be 89.5 degrees, i.e., our
view is only 0.5 degree from being edge-on.
Ephemeris for A-asteroid: Phase = Y - int(Y), where Y
= (BJD - 2457347.99603) / (4.50037 / 24), as
described in Rappaport et al 2016 (ref. at bottom
of this page). This keeps the presumed A asteroid
at phase ~ +0.12.
Ephemeris for
A-fragments: Phase = Y - int(Y), where Y = (BJD -
2457480.8083) / (4.4916 / 24). This keeps the
G6121 group of dips at close to phase zero.
Waterfall
Plot Overview
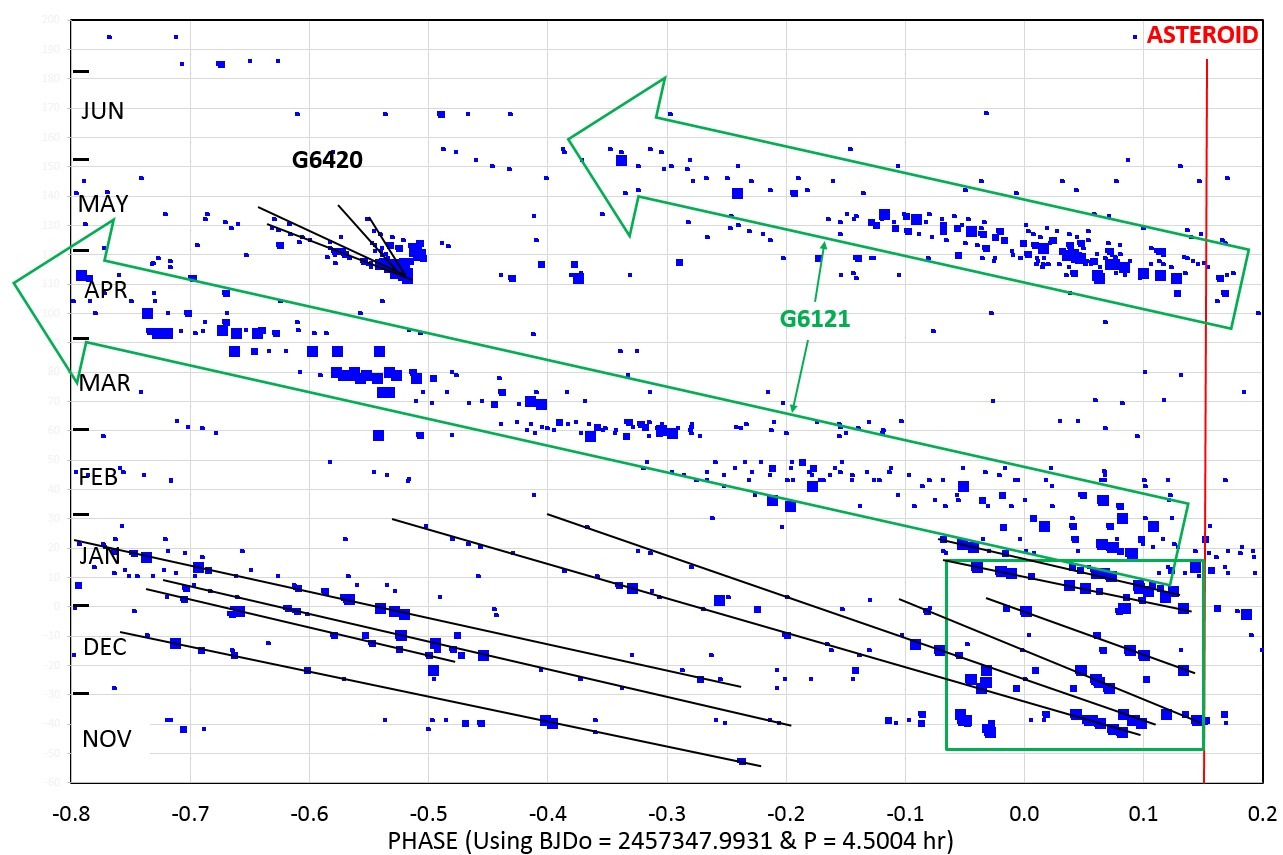
During November
and December most dips occurred at a phase
that we now assign to fragments that broke
off the asteroid and were active in
producing dust clouds for no more than about
2 or 3 weeks. During that time because they
were in smaller orbits than the asteroid
they drifted to earlier
phases (i.e., they appeared earlier than on
previous days). The phase shift during their 2
or 3 week of
activity was limited to about 0.2 phase units
(20% of the asteroid's orbit). This is shown
by the green box adjoining the red asteroid
crossing time line.
A few fragments were long-lived in
their activity, as seen on the left (during
November and December). In late January,
something dramatic changed: a large group of fragments
broke away and began a long-lived drift to
the upper-left
along a "corridor,"
and fragment
creation abruptly stopped.
The corridor
group (labeled "G6121") lasted for about 4
months, and starting in late May the fragment dust
clouds began a slow decline. By mid-July
they had all essentially disappeared.
Do the dips within the G6121 corridor
consist of long-lived drift lines? To answer this we need
to change the waterfall plot in a way that
shows drift lines better, and for the first
two months of the corridor.
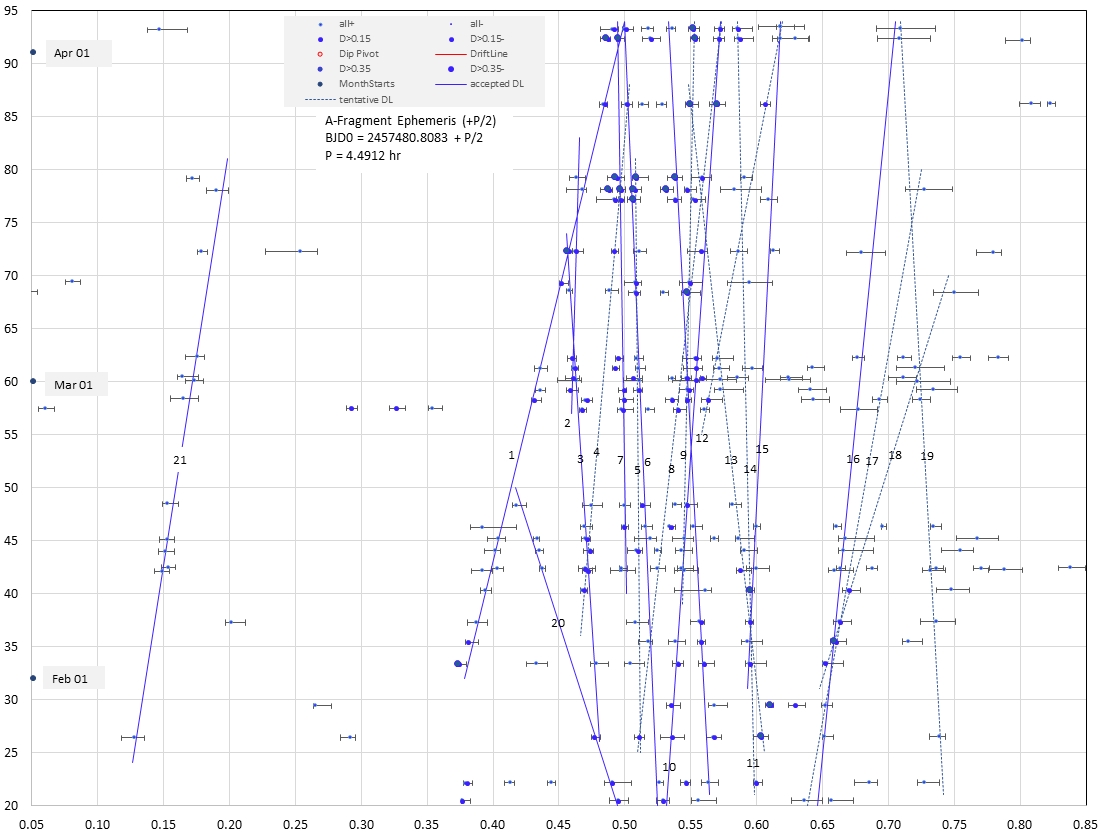
This is a detailed waterfall plot
using an ephemeris with the fragment period. It's
like rotating the previous waterfall
clockwise until drift lines are
vertical. The date coverage is only a
couple months, and it starts from the
transition date in late January. The
Y-axis is labeled in "day of year,"
beginning (at the bottom) with January
20, and ending
(at the top) with April 02. The phase
coverage is for the most active 80%
of an orbit. The symbols with bars
depict dip
features. The bars indicate
ingress and egress times (actually
~ 1/2 the length of those times).
There are 21 "drift lines" that
are either solid (believable) or dotted
(suggestive). Some dips last the entire
2.4-month interval shown, and others
last only 2 or 3 weeks. Dip depth
varies with date for each drift line,
and sometimes dip duration (ingress
plus egress times) also varies.
Studies of dip property versus time
are just beginning.
Orbit
Diagrams
Diagrams of fragment and A-asteroid location in
their orbits were being produced on an almost daily
basis during some of the 8 months of observing by our
team. We were hoping to see changes in "drift lines"
as fragments passed (i.e., lapped) their "mother ship"
asteroid. Such changes may have been seen, but the
light curves produced from our telescopes didn't have
sufficient SNR to be sure of these drift line changes.
This should be a goal for future observing seasons.
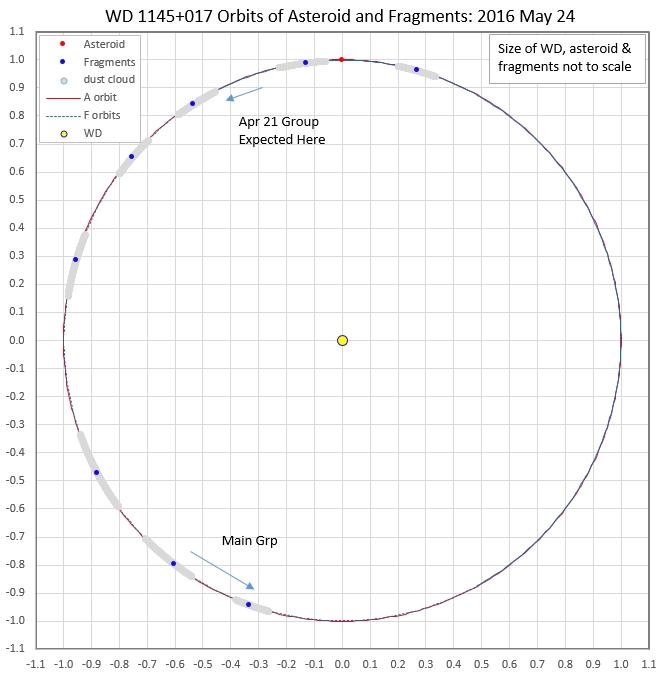
Illustration of a group of fragments (blue
symbols) that are actively producing dust clouds
(gray bands), that have caught up and overtaken
(i.e.,"lapped") their source, the asteroid (red
symbol). The two panels are 12 days apart (based on
actual observations). Since this coordinate system
is rotationally fixed to the asteroid the Earth can
be imagined being at the end of a line that rotates
clockwise. The size of the white dwarf is actually ~
1/2 of that shown (i.e., WD diameter ~ 1% of the
asteroid orbit diameter).

This is an attempt to show the actual
distance separating the asteroid orbit from the
orbits of the fragments. The diameter of the
asteroid is "to scale", or 1/2 the average
separation of the orbits.
Larger zoom, showing the asteroid (true to
scale) in relation to the range of fragment orbits.
We expect a uniform distribution of orbit distances
within the "range of fragment orbits." Approximately
25% of them will be close enough to the asteroid to
produce noticeable orbit changes (Petit
& Henon, 1986 and Rappaport, private
communication). The closest approaches will
produce an exchange of energy from the asteroid to
the fragment, thus slowing the asteroid by a small
amount (decreasing its orbit size slightly) but
speeding up the fragment by a significant amount
(and increasing its orbit size). An estimated 10% of
fragment orbit sizes will be increased to larger
than the asteroid's orbit, leading to a "horseshoe"
shape in the asteroid-fixed coordinate system. All
of the 25% of fragment orbit changes should be
detectable from the abrupt change in drift rate.
Such encounters, repeated many times, will cause the
asteroid's orbit to shrink over time. This will lead
to a smaller Hill sphere, which will increase the
gravitational gradient squeeze on the asteroid, and
produce a new episode of fragment creation. Another
cause for renewed fragment creation will be due to
the asteroid's shrinking orbit, where it will
encounter old (dead) fragments that will impact the
asteroid and start a new phase of fresh fragment
activity. In this manner the asteroid will
eventually undergo complete disintegration.
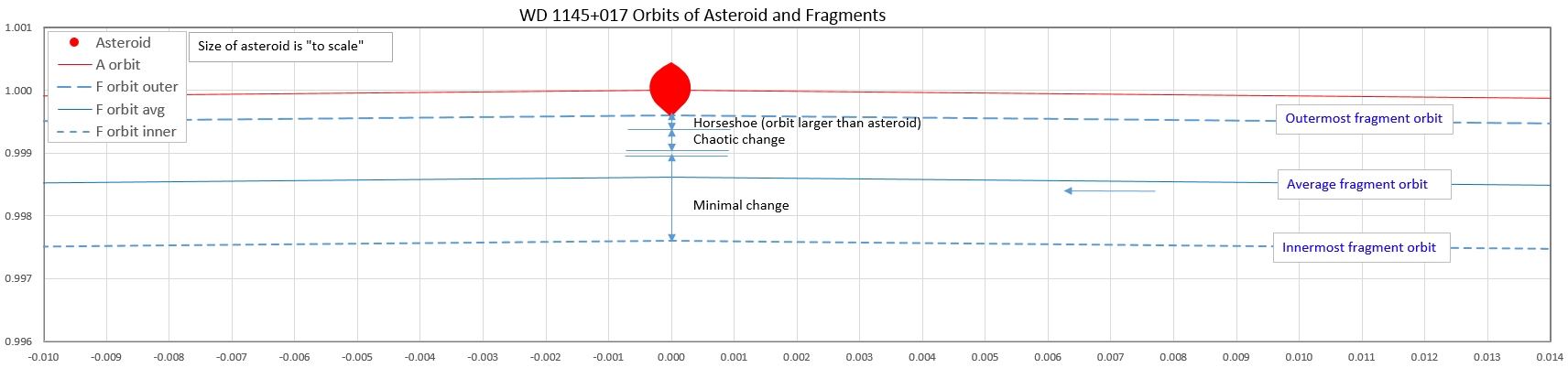
Detail of fragment orbit regions in relation
to their closest approach to asteroid. Three basic
regions are described by Petit & Henan (1986),
based on closest approach distance, d: 1) Rh < d
< 1.7 Rh, where fragment ends up in orbit larger
than the asteroid's orbit ("horseshoe"), 2) 1.7 Rh
< d < 2.6 Rh, chaotic orbits (some leading to
impact with asteroid), and 3) 2.9 Rh < d < 7
Rh, minimal change in orbit. The "horseshoe" orbit
gets its name from the path of the fragment in
asteroid rotation fixed coordinates; in non-rotating
coordinates the changed orbit is simply an ellipse
with periastron at the closest approach location and
apoastron at a distance farther from the WD than the
asteroid. The way such an orbit change occurs is
simply understood in terms of how much time the
fragment spends approaching the asteroid at one
speed versus how much time it spends receding from
the asteroid at a greater speed. Since the latter is
shorter, there's a net increase in speed due to the
approach. In other words, the fragment gains energy
as the asteroid loses the same amount of energy. The
fragment's change is observational noticeable after
one pass, whereas the asteroid's tiny orbit
shrinkage is not noticeable until after many such
fragment passes.
_________________________________________________________________________________________________________________________________________________
The rest of this web page is leftover from the
role it played in keeping the observing team
informed of what our observations were showing.
Casual raders may disregard it.
K2 Simulated LCs
This is a sampling of graphs showing our
ground-based light curves smoothed with a 30-minute
box function that results in a simulation of what the
Kepler spacecraft would have observed in its 30-minute
cadence observing mode.
Traditional Abstract
WD 1145+017 (hereafter WD1145) is going to be the
prototype for a new category of variable star. These
will be white dwarf stars with asteroid-size
planetesimals orbiting so close to the star that the
strong gravitational gradient is "putting the squeeze"
on the asteroid (i.e., with the asteroid's "Hill
sphere" shrinking to the size of the asteroid). WD1145
is a white dwarf star about the size of Earth, with a
mass of 0.6 times the sun, being orbited by an
asteroid every 4.5 hours with an orbit radius of
0.0054 a.u.. This is close enough for fragments
to break off the side facing the star (the L1 point),
and enter orbits slightly smaller than the asteroid.
These fragments then produce dust clouds that obscure
the star when they orbit in front of it, causing brief
star fades similar to exoplanet transits. Since last
November WD1145 has had at least a dozen such
fragments with a variable production/destruction of
dust such that during each 4.5-hour orbit there are
about a dozen fade events that are deep enough for
amateur observers to measure (i.e., up to 60% fade, or
~ 1 mag). These fade events last ~5 minutes,
typically, and their depth and shape change slightly
with each orbit.
In addition to these daily dynamics there are
month timescale changes, and also year timescale
ones. For example, Kepler's mid-2014 discovery
observations occurred during a "quiet phase," and
ground-based follow-up observations by professional
astronomers 8 months later showed that the quiet
phase consisted of brief fade events that could be
as deep as 40%. Later, in the summer of 2015, a
collision must have occurred that caused many new
fragments to break off the asteroid and enter
slightly smaller orbits (via the L1 path), and these
caused an increase in fade activity (as I discovered
last November). The fade activity reached a peak in
2015 December, being ~ 15 times greater than during
the Kepler K2 discovery observations. Our amateur
observations have shown that we are now in a
decreasing activity phase, perhaps leading to the
same quiet phase that existed during the K2
observations 1.7 years ago.
Another collision could produce a new outburst of
active fades, and this could happen at any time;
sampling theory suggests that such activity
outbursts could occur at several-year intervals. To
monitor this using professional telescopes would be
prohibitively expensive, and since it is easily
monitored with amateur telescopes I urge all
amateurs (with exoplanet transit experience), and
with telescopes at least as large as 11" aperture,
to consider monitoring this star on a regular basis
(similar to the way AAVSO observers are monitoring
KIC 8462852 in hopes of discovering another big fade
event). AAVSO expressed interest in accepting light
curve data submissions for WD1145, but so far there
is no provision for this. The ETD is already ready
to accept submissions, but their new set of web
pages have not yet provided full support for
English-speaking observers. I am attempting to form
a group for semi-global coverage; I need volunteers
from longitudes in Asia. Not only will WD1145 be an
interesting star to monitor and study for many
years, it will be known as the founder star, a
prototype, for a new variable star category: "white
dwarfs with dusty transits." Large professional
survey telescopes will surely reveal the presence of
more of these stars. They will be attractive to
amateur observers and professionals alike, not only
for their daily and yearly changes, but because they
offer a glimpse of what our solar system will be
like in a few billion years. Indeed, when our sun
becomes a white dwarf it may become a WD1145 type
system with the Earth becoming an analog to the
WD1145 "asteroid." Imagine, the atoms in our bodies
may some day contribute to dusty clouds that produce
fades seen by alien observers like you and me in
that distant future time.
This web page is devoted to a photometric
monitoring of WD 1145+017 by observer Bruce L.
Gary, starting 2016.01.27 (i.e., after submission
of a paper based on earlier amateur observations).
Starting in 2016 April I recruited a few other
amateurs (the "longitude team") to contribute, so
those LCs are presented here. Another web page
with those earlier observations, from 2015.11.01
to 2016.01.21 by a team of 4 amateur observers
that I coordinated, will be converted to public
domain status after publication of our article in
MNRAS (soon). In the meantime you can view our
arXiv article here: link.
Traditional Introduction
This white dwarf star was discovered to exhibit
transit behavior, as described by Vanderburg et al
(2015) using Kepler K2 data during 2014 Jul/Aug/Sep.
K2 was limited by exposure times of 30 minutes, so
shorter fade events were greatly smoothed and it was
not known if the changing light curve amplitudes
(within the range 0 to 2%) were due to long transits
that changed depth or shorter ones that were more or
less numerous, or some combination of both. At least
the K2 observations showed a clear Lomb-Scargle
periodogram signal with a period of 4.4989(1) hours.
An attempt was made to clarify the situation using
follow-up ground-based observations with professional
telescopes during 2015 Mar/Apr/May. The results of
these ground-based observations were included in the
Vanderburg et al (2015) paper, where it was speculated
that at least one planetesimal was disintegrating and
producing thick dust clouds that transited the white
dwarf (WD). Croll et al (2015) also arranged
for ground-based follow-up observations with
professional telescopes during 2015 May. Both of these
ground-based observing projects found isolated fade
events that were short, and much deeper in depth than
seen by K2, but many basic questions remained about
what model could account for disappearing and
reappearing fades (at different times than expected
from the K2 A period). The mysterious nature of
WD1145 was made public in late 2015 October by
simultaneous postings of the two above-cited articles
at the arXiv web site. This created an opportunity for
amateurs to begin observing WD1145.
Amateurs Join the Fun
The best observing season for WD1145 is centered on
mid-March, when in 2015 the two groups of professional
astronomers used professional telescopes for follow-up
observations. In early November, when I began
observations, WD1145 could only be observed for ~ 1.5
hours and always at low elevation angles (high air
mass, especially bad for a blue star). In spite of
this handicap my first observation, on 2015 Nov 01,
showed that this 17th mag WD was feasible for
observation by amateurs with telescope apertures of
only 14". The original purpose was to help Dr. Bryce
Croll prepare for professional telescope observations
in 2016 February, which he indicated would be useful.
On Nov 8, when I measured a 0.4-mag fade event, I
began to suspect that amateurs could provide
scientifically useful observations, so I began
recruiting other amateur observers to help (starting
with Paul Benni, Nov 15, and Jerry Foote and Tom Kaye,
Nov 24). By late November it became obvious that
WD1145 was very active, and that our amateur
observations were potentially valuable by themselves.
Indirect indication of this came when we learned, in
late November, that Dr. Croll had arranged for
observations with two professional telescopes, 2.5
months ahead of his original plan. Actually, we had
more direct confirmation of the value of our
observations from frequent e-mail exchanges with
professional theoretical astronomer Dr. Saul
Rappaport, who had maintained contact with us since
October (when he suggested to me that Dr. Croll might
appreciate our help). It was Dr. Rappaport's
enthusiastic encouragement that caused our observing
efforts to go into "high gear" in mid-November. Dr.
Croll's appreciation of our observational results,
which he could monitor from a web page that I
maintained, became more apparent when he told us of
plans to present our data at the Extreme Solar Systems
III conference in Hawaii, 2015 Dec 4. He also
presented our data at the 227th Meeting of the AAS, in
Florida, 2016 Jan 4-8. After Tom Kaye and I persuaded
Dr. Rappaport that our fade events were drifting to
earlier times with respect to the K2 A-period, Dr.
Rappaport came up with a clever model involving an
asteroid-sized planetesimal that had migrated so close
that its "Hill sphere" had shrunk to the size of the
asteroid, and any fragments breaking off
(preferentially at the hot, L1 end) were free to
wander into an orbit slightly smaller than the
asteroid, and this accounted for the drifting that we
amateurs had documented. This eventually led to a
15-page manuscript that was submitted to MNRAS
(on 2016 Jan 27); it also appeared at the arXiv web
site (astro-ph.EP) on 2016.02.02: http://arxiv.org/abs/1602.00740.
Why This Separate Web Page
Another web page has a detailed display of amateur
observations from 2015.11.01 to 2016.01.21:
http://www.brucegary.net/WD1145/
It was in the public domain until one of the
professional astronomers on our MNRAS paper requested
that it be PW protected, to prevent other professionals
from knowing what we were doing. I consented,
reluctantly, and when the MNRAS paper with responses to
reviewer comment is finally accepted by MNRAS (probably
2016.03.07) I will place that web site back into the
public domain. Until then, my observations since the
last one used in the MNRAS paper are being posted at
this web site, which begins with a 2016.01.27. These
observations document a dramatic reduction of fade
depths (i.e., dust production by a dozen or more
fragments still in orbit). It now appears that something
happened last August to increase activity to ~ 15 times
greater than 1.7 years ago (during K2 observations), and
also much greater than found using professional
ground-based telescopes last Mar/Apr/May; the "activity
level" peaked in early Dec, and has been declining ever
since and may be on-track for returning to the K2 level
of activity sometime this year.
Remaining Questions
Many questions remain about WD1145. Foremost is
whether Dr. Saul Rappaport's clever idea is right,
that the asteroid's Hill sphere has shrunk to the size
of the asteroid body, forcing it into a football shape
with the star side coinciding with the L1 location
that produces fragments that break away into orbits
corresponding to the location of the L1 distance to
the star. (Actually, due to gravitational pull during
the departure phase the fragment should end up in an
even smaller orbit than corresponding to the L1
distance.) Another question relates to the drift
lines: why don't they all have the same drift rate?
Their variance requires that the fragments have
slightly different orbit periods; could this be
explained by them having a variation of velocity
component in the for/aft direction as they leave L1?
If fragments break off from L1 in groups, can their
different drift lines be used to infer when the
break-off event occurred, by projecting their set of
drift lines backwards in time to a date when they
converge? Or do the fragments break away at random
times, not in groups but as single fragments? Do the
fade depths vary with wavelength in a way that can
provide a solution for dust size distribution? How
long can a fragment produce dust? What are the
patterns for fade depth variations, and how can these
be accounted for by variable dust production and loss
rates? How often are there episodes of increased
fragment creation and dust transiting activity? During
a 1.7-year interval we've seen one such event. Could
this have been pure luck? Do we have a correct orbital
period for the asteroid, which relies mostly upon the
3-months of K2 data (when WD1145 was inactive), and
which is only partially supported by the amateur
observations of the past 4 months? Both solutions,
using Lomb-Scargle periodograms, could be influenced
by the drift behavior of fragments and their turn-on
latency after breaking off the asteroid. During the K2
observations were there very few fragments, and only
the L1 part of the asteroid was "glowing" with dust
that stayed near L1, thus allowing for a determination
of the asteroid period? This is one way to reconcile
the absence of an A-period signal from data taken in
the last month. What about the other periodicities
derived from the K2 LS analyses? Do they correspond to
other asteroids in slightly larger orbits that only
infrequently produce fragments? Are all "dusty" WDs
destined to go through a phase of being like WD1145,
lasting until all planets and asteroids have
disintegrated by Rappaport's L1 break-away mechanism?
Presumably, each planetesimal, or asteroid, will
disintegrate when it migrates close enough to its WD
star; how long will the disintegration process last?
And are there intervals with no dusty transits between
times when a succession of asteroids migrate close
enough to begin their disintegration? How long will
this activity last? Are we simply very lucky to be
observing a rare event, or will WD1145 be active, with
the level of variability already observed, for
centuries and millennia?
How Will Pro's Use Amateur Monitoring Data?
The most important use for future monitoring
observations of WD1145 will be to know when the next
outburst of fragment creation occurs. With the
announcement of this next event (possibly later this
year, or next year, etc) an intensive program of
observations will begin with several goals in mind. We
need to determine how to make full use of the pattern
of drift lines. At this time we don't even know why
there is such a large variance of drift rates, so this
will be one of the first questions treated by the new
observations. The drift lines should converge
backwards in time to the date of the creation of the
dozen or more fragments (assuming fragments are
created in groups). The convergent point should also
allow for a determination of where the asteroid is in
terms of A-period phase. We think it is at ~ +0.10,
but this is still uncertain. This will also help in
refining the A-period, which is important for many
other goals for understanding WD1145. Documenting an
outburst of activity from its inception will permit a
determination of turn-on latency statistics. We
suspect that a newly-created fragment may not create a
dust cloud for several days, or maybe weeks. Fragments
are known to undergo reduced activity (dust
production), and these times of reduced activity can
be used to model dust destruction rate as well as
production rate variations. This information can be
used for constraining models for the dust cloud's
production and loss. The total longevity of a
fragment, coupled with its activity history, can be
used to determine fragment size. Observations are
currently underway to determine fade depth versus
wavelength, from B-band to near-IR, for the purpose of
deriving a particle size distribution (PSD). Since the
activity level is now very much lower than during the
main outburst phase (last November/December), these
observations may not be able to provide the required
data for a PSD solution. The next outburst will
provide a better opportunity for these observations.
Even amateur observations should then be useful for
this purpose. PSD is needed for several things, such
as yielding a more accurate fragment size and modeling
radiation pressure effects on particle movement away
from (and toward) the WD star.
Staying in Public Domain
In the past I've observed for pay at the rate of $20 /
observing session hour, or $120 / complete observing
session (depending on whether the target was a moving
asteroid versus a star, and how much analysis was
required). However, for WD1145 I've decided to just
observe it when I feel like it, and not charge for
making the results publicly available. Therefore,
this web page isn't PW protected, so I welcome anybody
to take from it whatever they want. I only welcome
e-mails from friendly astronomers; if you're one of the
others then just take what you want from this web page
and mention my name in the Acknowledgement section of
your publication, if appropriate. For me the fun is
observing, and figuring things out.
Summary of
Results
Many dips are still present per orbit, as of
2016 Mar 01, but they are concentrated at phase ~
0.5 (different from Nov & Dec, when there were
fades at all phases but concentrated near phase
zero). A K2 simulation shows fade depths within the
range 8% to 24% (with shape sometimes resembling K2
mission findings). The activity level is decreasing
slowly (good luck for 2016 observers!). I think
activity level rose from the low level observed
during K2 to the high December, 2015 level starting
in late July and mid-August (based on convergence
times of fade drift lines). This is when an old
(dead) fragment may have had a collision with the L1
end of the asteroid, prompting a new set of fragment
breakaways. Sampling theory suggests that such
renewals of activity may occur at 2 or 3 year
intervals.
Data file: data
(this is the 2016.12.19 version. Plz check
for revised versions later.)
Activity Level
"Activity Level" for a
date is defined as "the area under the curve" for
one orbit on that date. It is calculated by summing
the area of all dips needed to fit a LC for that
date. Note, the area under one dip is 0.79 x depth x
(tau1 + tau2) / 4.5 hrs.
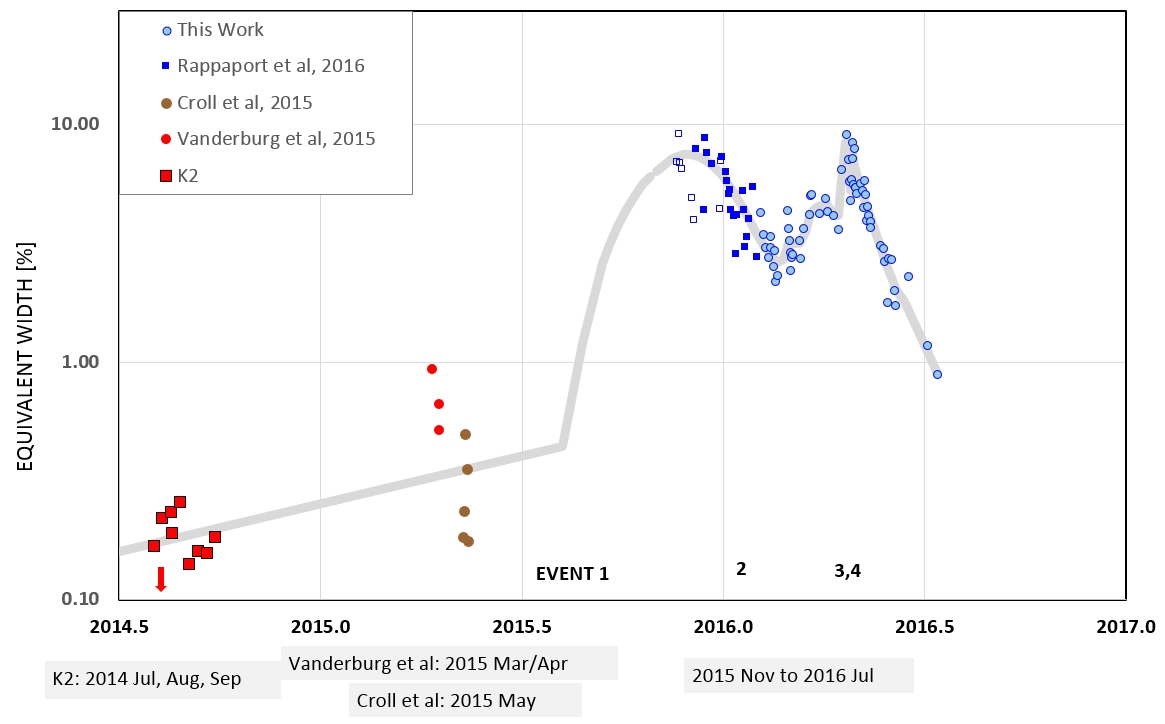
The EW (equivalent
width, or total area under all dips) is currently
0.044 vs. 0.00146, the median before event A
(~2015 Sep 1).
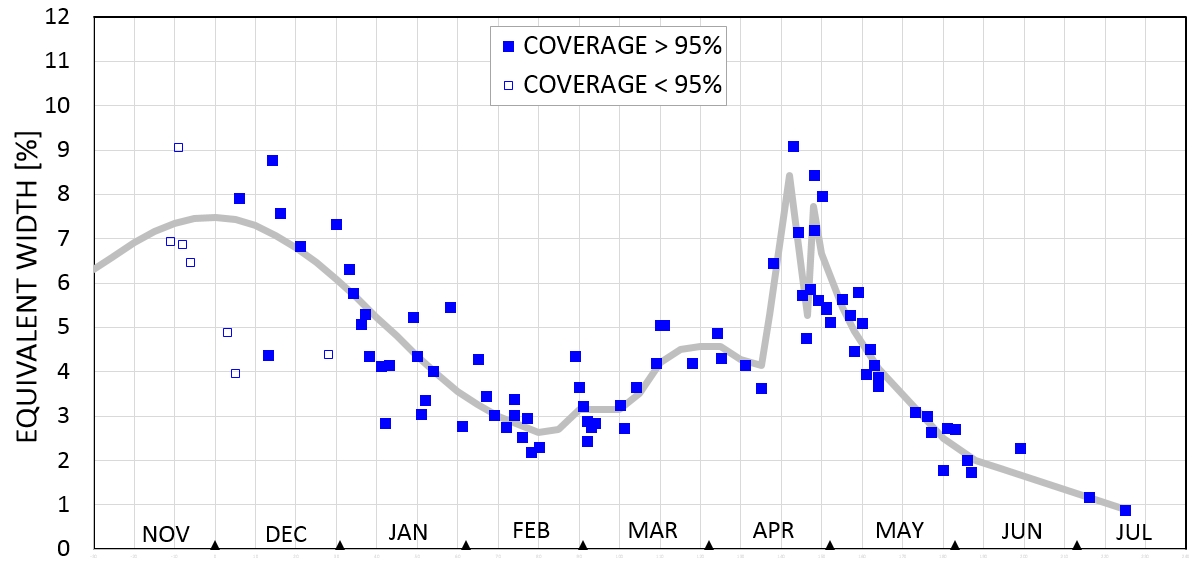
Total fade
"equivalent width" is the sum of all fade events
(depth × (tau_ingress +
tau_egress)), during an
orbit. Open squares are lower limits due
to incomplete orbital coverage; small squares are
attempted adjustments of open squares to estimated
complete coverage value. The model takes into
account a subjective estimate of the incomplete
coverage for Nov/Dec data. The smooth model
overlooks what appear to be real changes on
smaller timescales. There appears to be a jump in
activity on 2016 Apr 14 or 15, with a peak of
activity on Apr 21, then a decrease that has
lasted for 3 months.
The activity level was trending downward with
date from early December to mid-February, as
illustrated by the following two LCs:
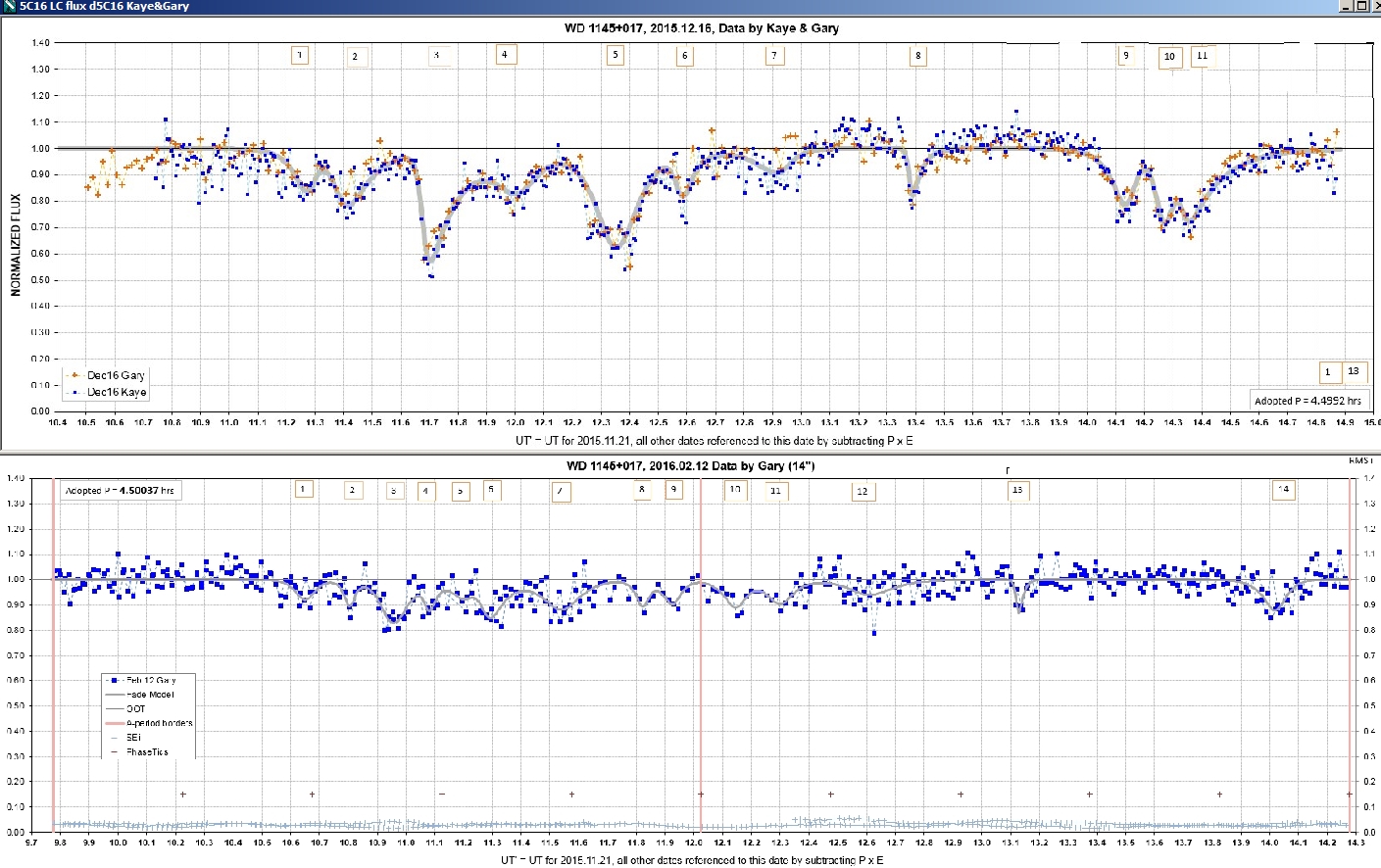
The number of fade
events didn't change but their depths did!
Starting in late February the depth of fade events
(dips) increased.
Waterfall Plots and Speculation
Note: The
following plots have time increasing upward (so
these could be "water-rise plots").
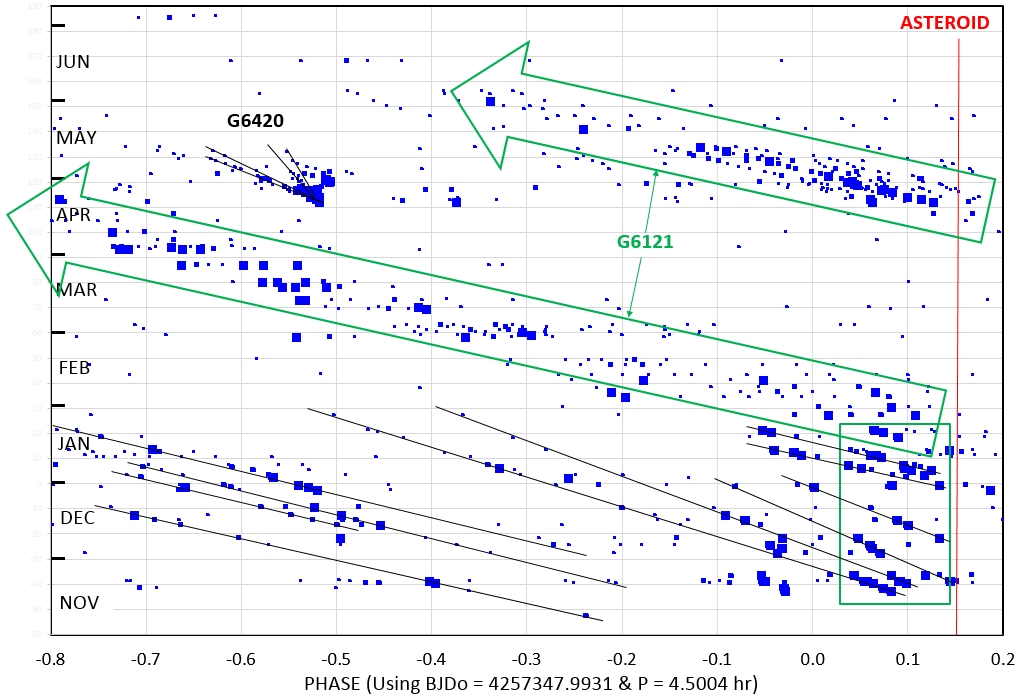
This is an
overall location of dip features during the past 5
months using the asteroid's A-period ephemeris for
defining phase. Date is defined as days since Nov
30. The vertical red line is identified with the
asteroid. The rectangle of activity (centered on
phase +0.10) has a period that agrees with the
Kepler A-period. Symbol thickness is proportional
to dip depth. (As of 2016.06.22)
I speculate that until ~ Jan 21 most fragments
that left the asteroid "mother ship" were small
enough to "turn-on" almost immediately but then ran
out of mass and died within a few weeks, still close
in phase to the asteroid source. This allowed them
to show the A-period of their source asteroid.
Imagine that the asteroid is located at phase +0.15,
and the drift lines represent fragments breaking off
the L1 end of the asteroid at dates corresponding to
the intersection of the backwards-in-time projection
of the drift line to the asteroid, at phase +0.15
(vertical red line). The first drift line would then
correspond to an origin of 2015 Oct 12. The last
breakaway event is indicated by the large group of
asteroids that occurred on ~ 2016 Jan 21. This group
of fragments was apparently large enough to remove
"pressure" from the squeezing effect of the
gravitational field gradient at the L1 end of the
asteroid, so fragment breakaways stopped on that
date.
I propose that the size distribution of fragments
for the Jan 21 breakaway event included more large
fragments compared with the earlier breakaway
events. Since the surface area to volume ratio is
inversely proportional to fragment size we can
expect a lower level of activity (dust production
per unit mass) immediately after the breakaway time,
corresponding to a longer turn-on latency; but once
turned on they should remain active longer and
should turn-off much later than their small fragment
counterparts. The Jan 21 event must have yielded
many large fragments that didn't turn-on in a
significant way for ~ 2 months, about mid-March. So
far, this group of fragments has remained active for
almost 2.7 months, and may continue to be active for
many more. (The fact that some drift lines don't
extend all the way back in time to the asteroid's
location, at phase +0.13, can be explained by
assuming a fragment can remain "dormant" for many
days, or weeks and months depending on size, before
it begins to produce dust clouds.)
Note how a fragment's dip depth varies with time.
This constrains modeling of dust production and loss
timescales. Presumably dust loss rate is constant
but dust production rate is variable, accounting for
variable dip depth vs. time for each fragment. The
turn-on latency may be a good indicator of fragment
size, with longer latency corresponding to larger
size.
The large breakaway event of 2016 Jan 21 exhibits a
drift rate (with respect to the asteroid) of -3.0
[min/day]. This leads to a complete phase shift in
90 days; that's the time it takes for the fragments
to "lap" the asteroid. This group of fragments is
predicted to pass the asteroid on Apr 20. If the
asteroid's gravitational influence is strong enough
to change fragment orbits then we should see a
change in dip pattern starting on that date. For
example, the drift lines should begin to diverge
more, and take on a less-steep slope (closer to zero
than -3.0 min/day). Speculating further, if orbit
disruption does occur on about Apr 20 we might
expect that one of the fragments with a disrupted
orbit could impact the asteroid 90 days later, and
cause a large number of new fragments to be
produced, causing dip activity to increase
dramatically. Apr 20 plus 90 days is Jul 19; that's
unfortunate because WD1145 will be closing-in on the
sun then, being observable for < 1 hour per night
before sunset.
Consider the Apr 20 close approach of the asteroid
with many fragments. They will be close together for
something like a week. Therefore, there will be an
outward gravitational tug during the week long
passage. In other words, the fragments will have a
larger orbit, and still approximately circular
(because their proximity lasted many orbits). Since
the fragments are in a new, outer orbit, their lap
interval will increase to more than 90 days. After
many such passes, at 90-day intervals initially, but
stretching to longer and longer intervals, the
probability of a collision increases. Collisions can
be the trigger for a new phase of fragment release
and dust cloud dip activity. How long can such a
scenario endure? Until the asteroid is totally
destroyed!
What a neat solar system!
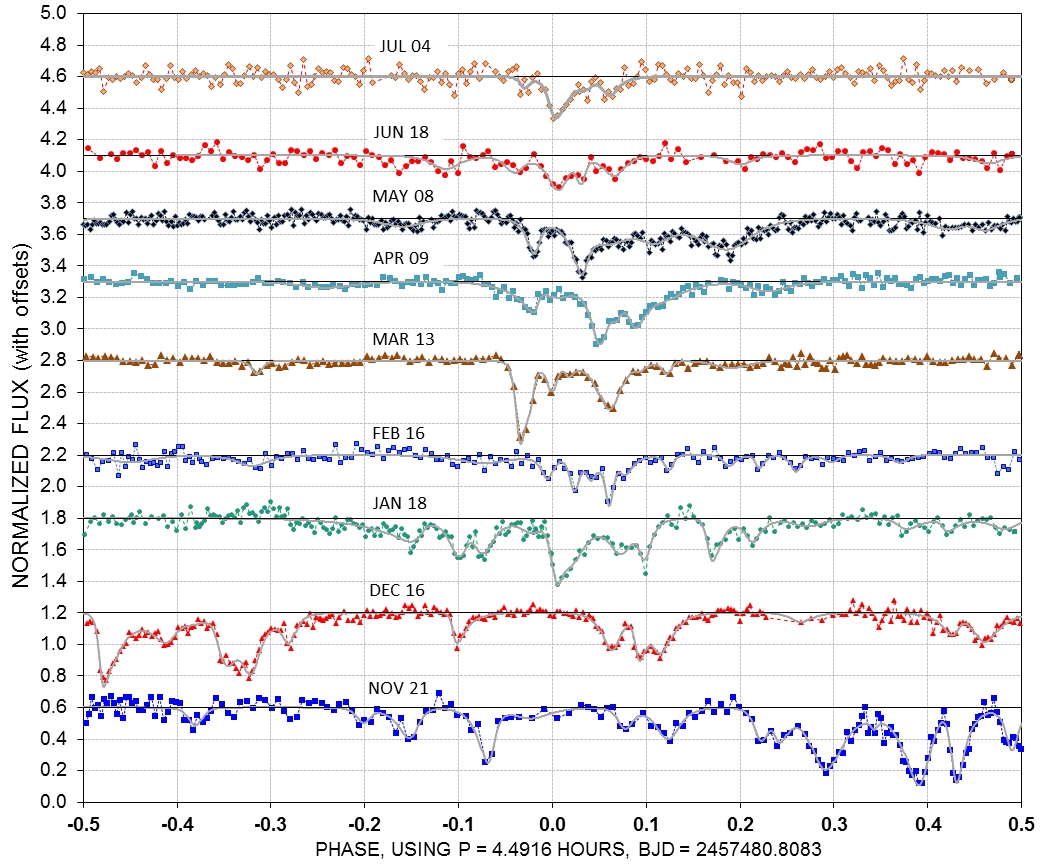
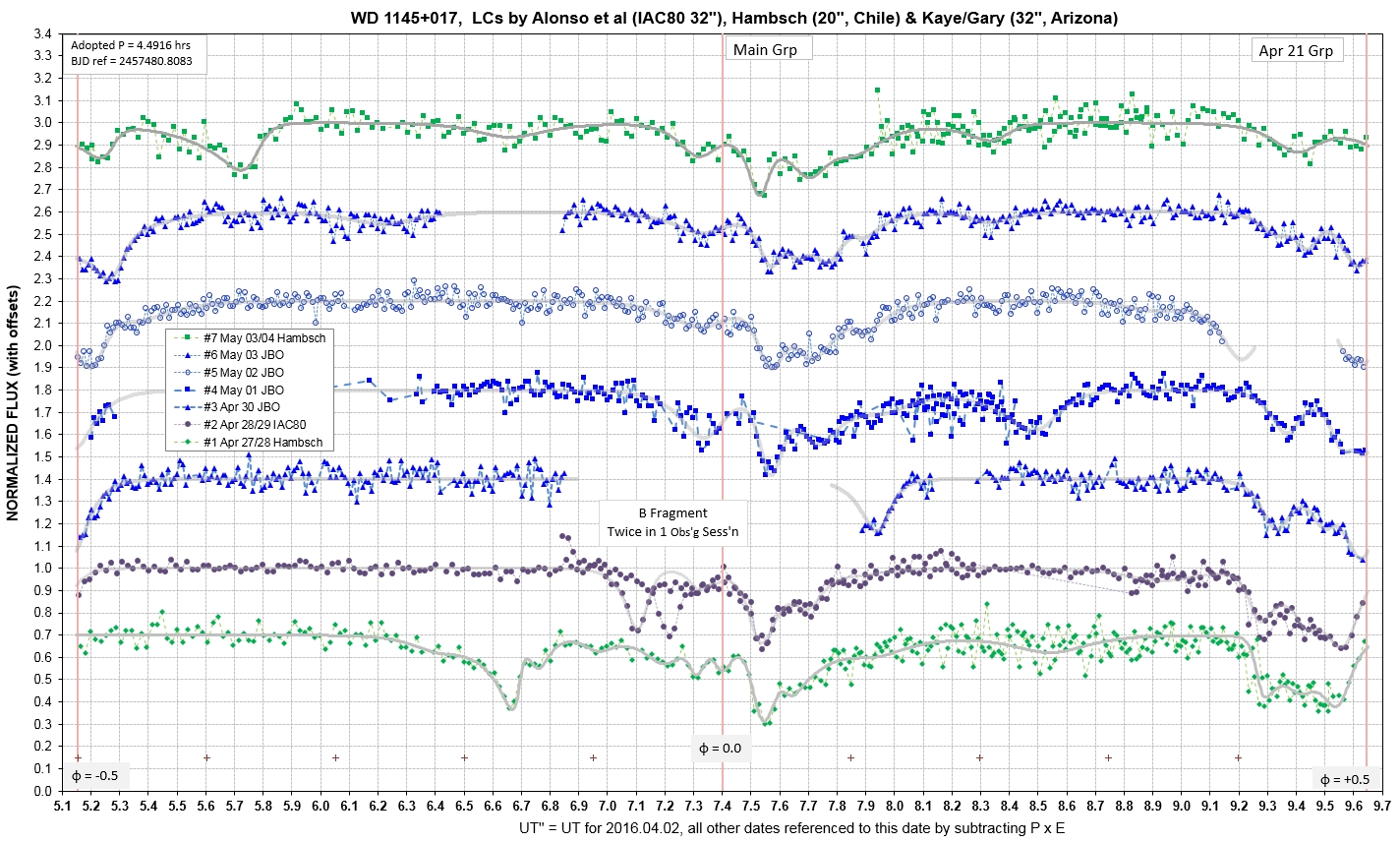
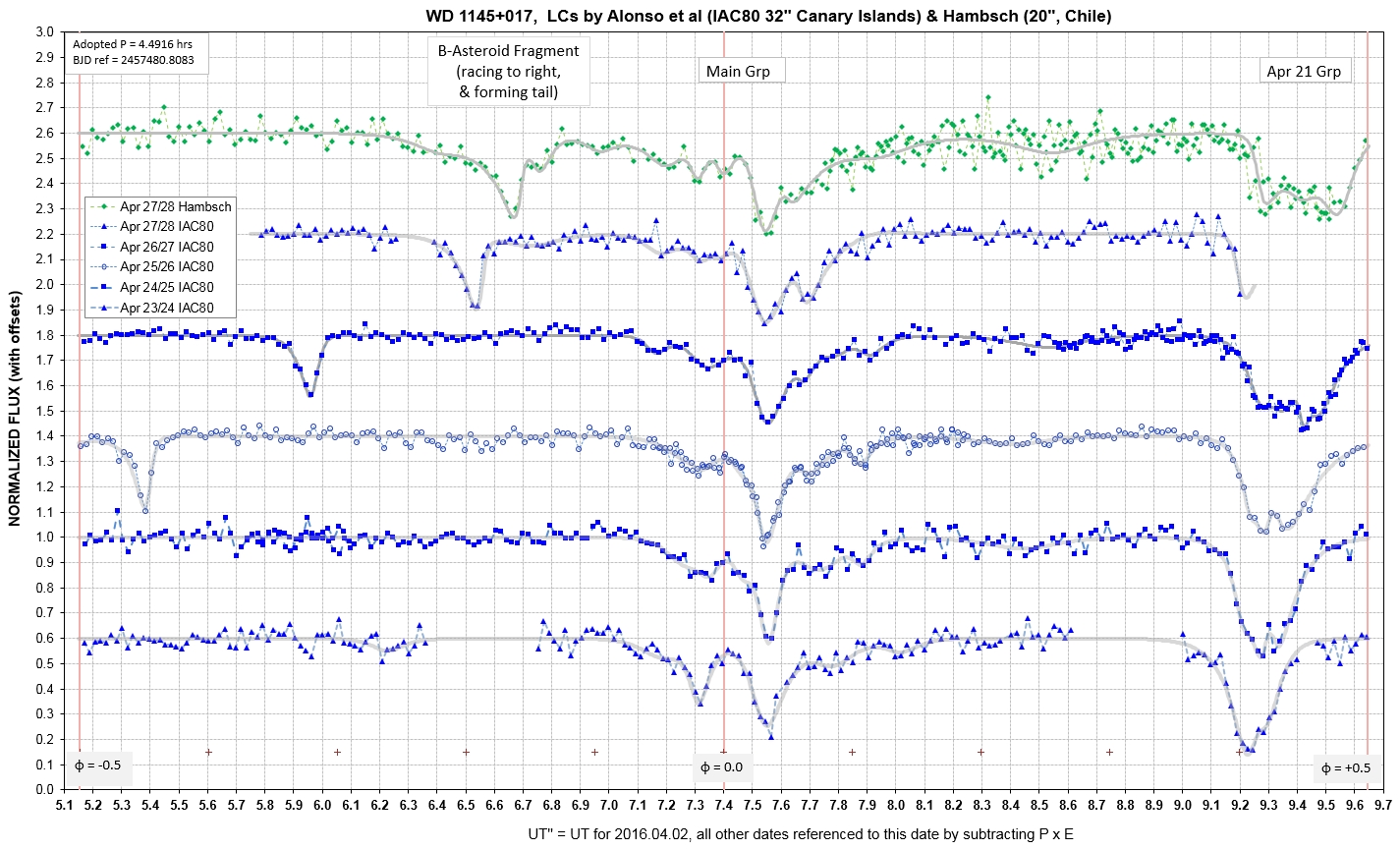
These are
traditional waterfall plots (except that I show
time moving upward).
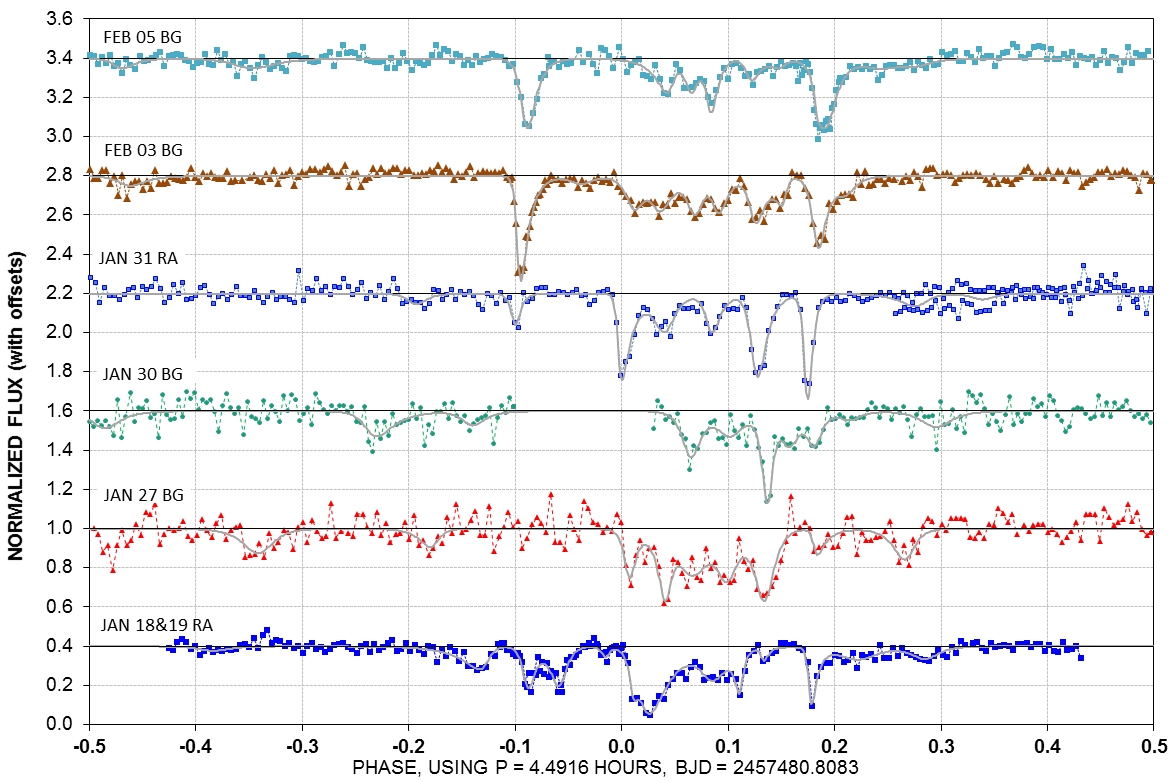
During the
G6121 "transition" this is how the LCs stacked-up
(using the A-fragment, G6121, ephemeris).
Waterfall Plots
Waterfall plot showing 80%
of an orbit (i.e., phase) for a 2.4-month
date interval (starting with the Jan 21
transition date). The 21 "drift lines" are
solved for using an objective protocol
(maximum of sum of depths encountered vs.
slope, for continuous sampling of phase
offset hinge points). The 10 solid line
drift lines are what I would call
"acceptable" (a subjective assessment) while
the 11 dotted lines are merely "suggestive."
(I have some feedback from a couple
professionals who are skeptical about such
drift lines. They essentially say "Can you
prove that the dip associations belong
together?" My answer: "No, of course not,
but be reasonable!")
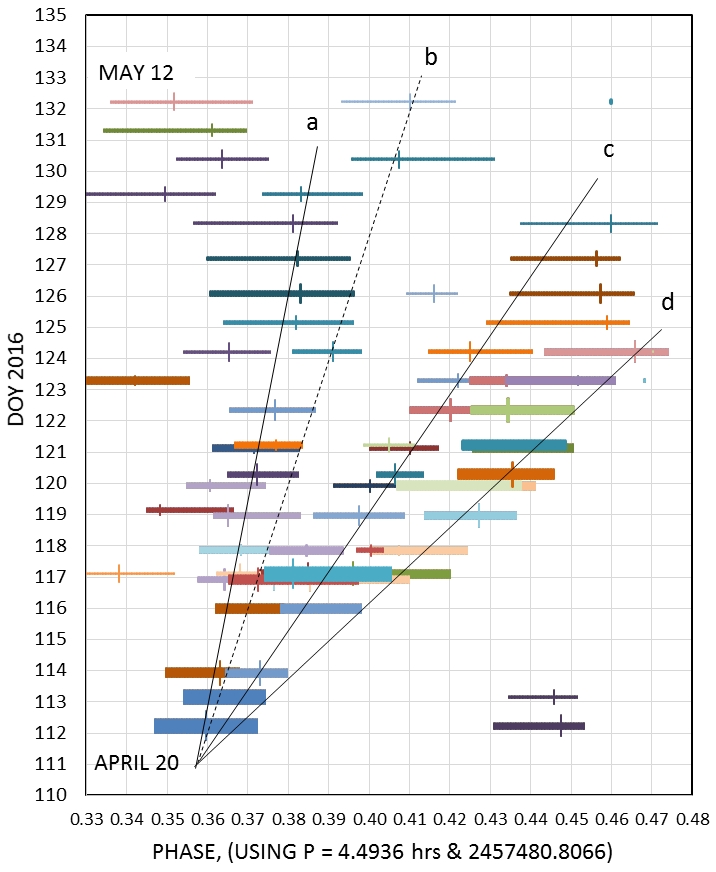
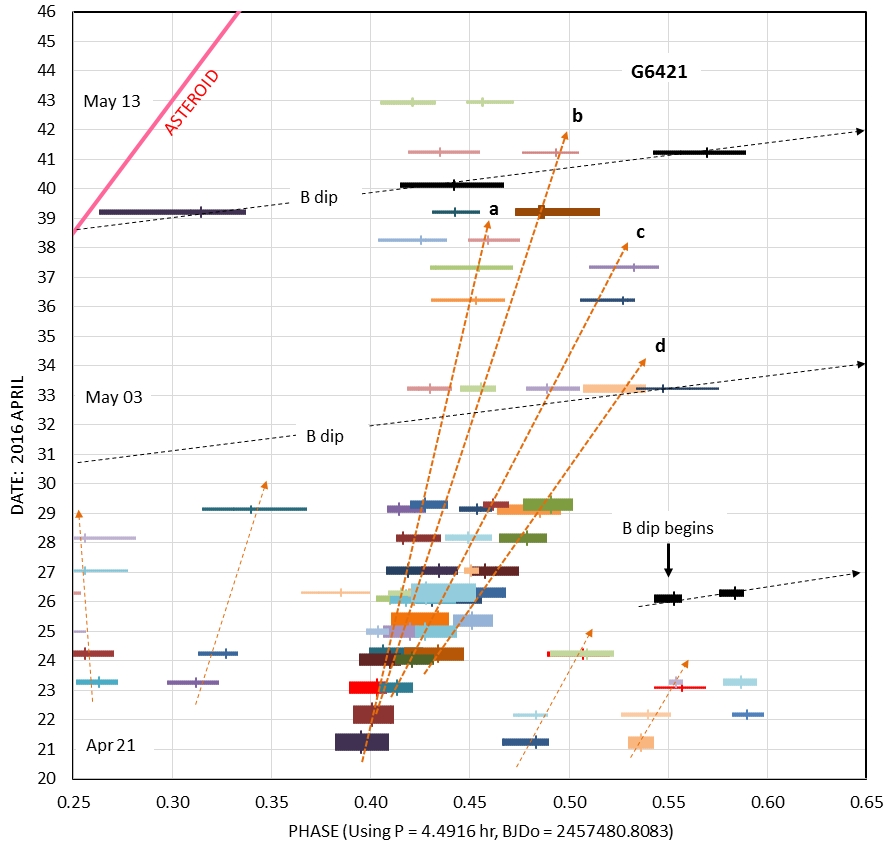
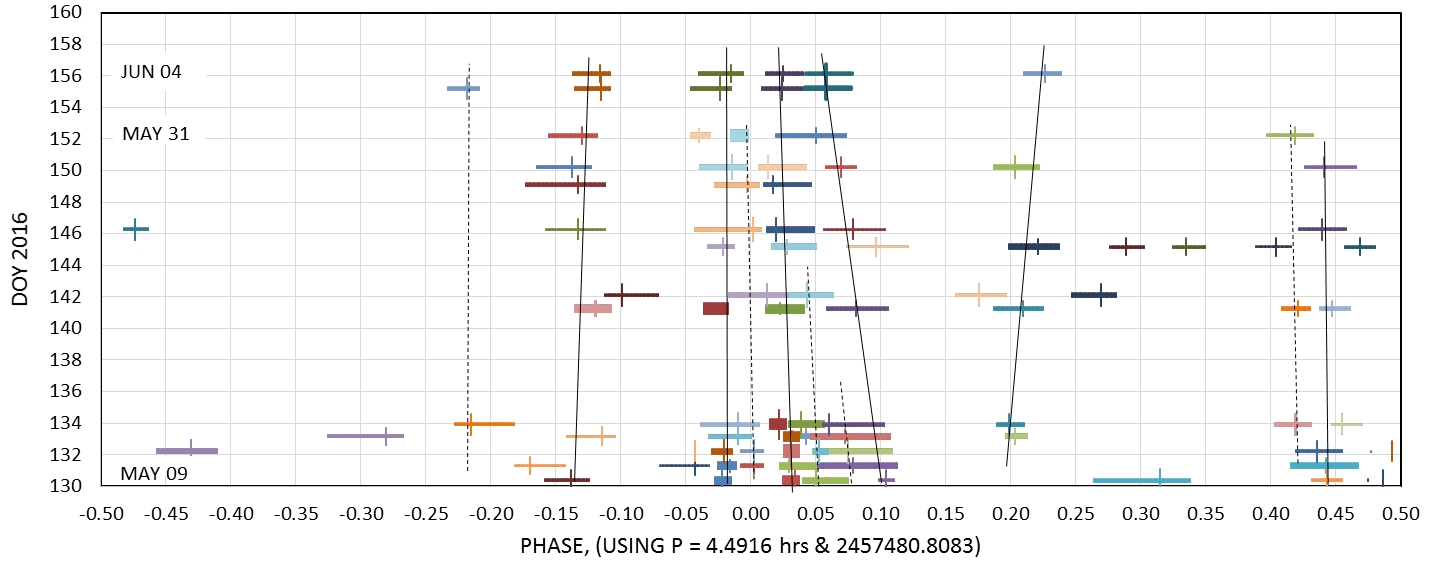
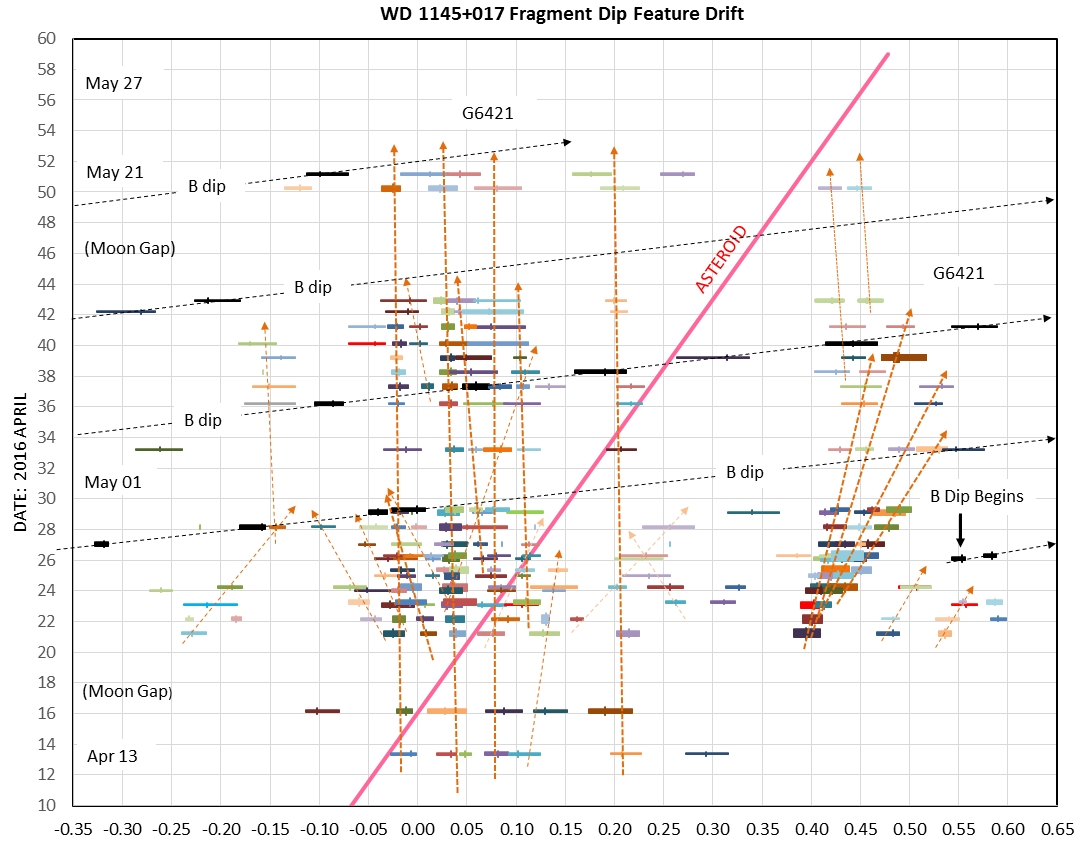
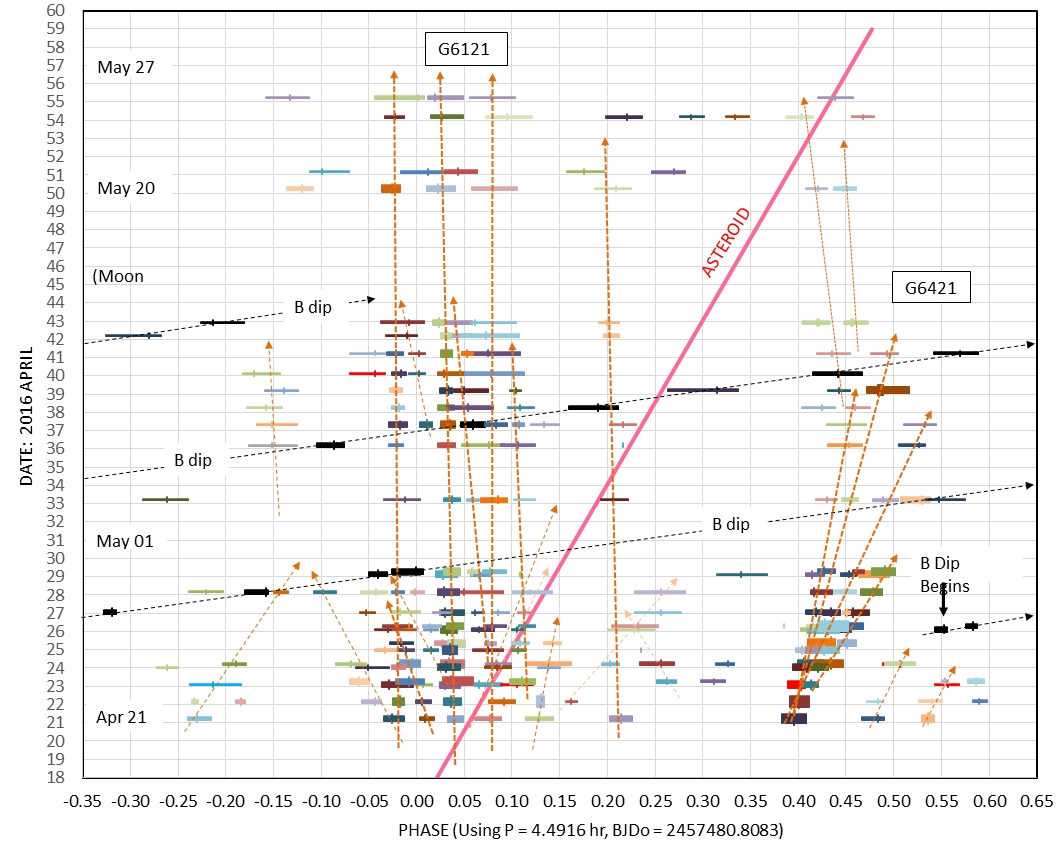
This 2016
April waterfall plot adopts fragments period of
4.4916 hrs. The red line on left is my provisional
estimate for the asteroid's phase location (using
P = 4.50037 hrs). Fragments that pass the asteroid
(i.e., lapping it) will move upward to the red
line and then veer to the right with a slope
greater than the red line (i.e., corresponding to
the fragment being in an orbit larger than the
asteroid). Two fragments that have passes the
asteroid appear to have reversed drift direction,
and are now drifting to the right at a rate
similar
to, or greater than, the
asteroid. A fragment that turned-on at location
"Apr 21 / phase +0.39", is moving the right. Since
it was far from the asteroid when it appeared it
may have been caused by a collision of two
previously dead fragments, exposing 3 fragments
with fresh surfaces for producing dust clouds
(lasting 2.5 weeks).
Below are a few old waterfall plots, showing
behavior, up to Mar 20.
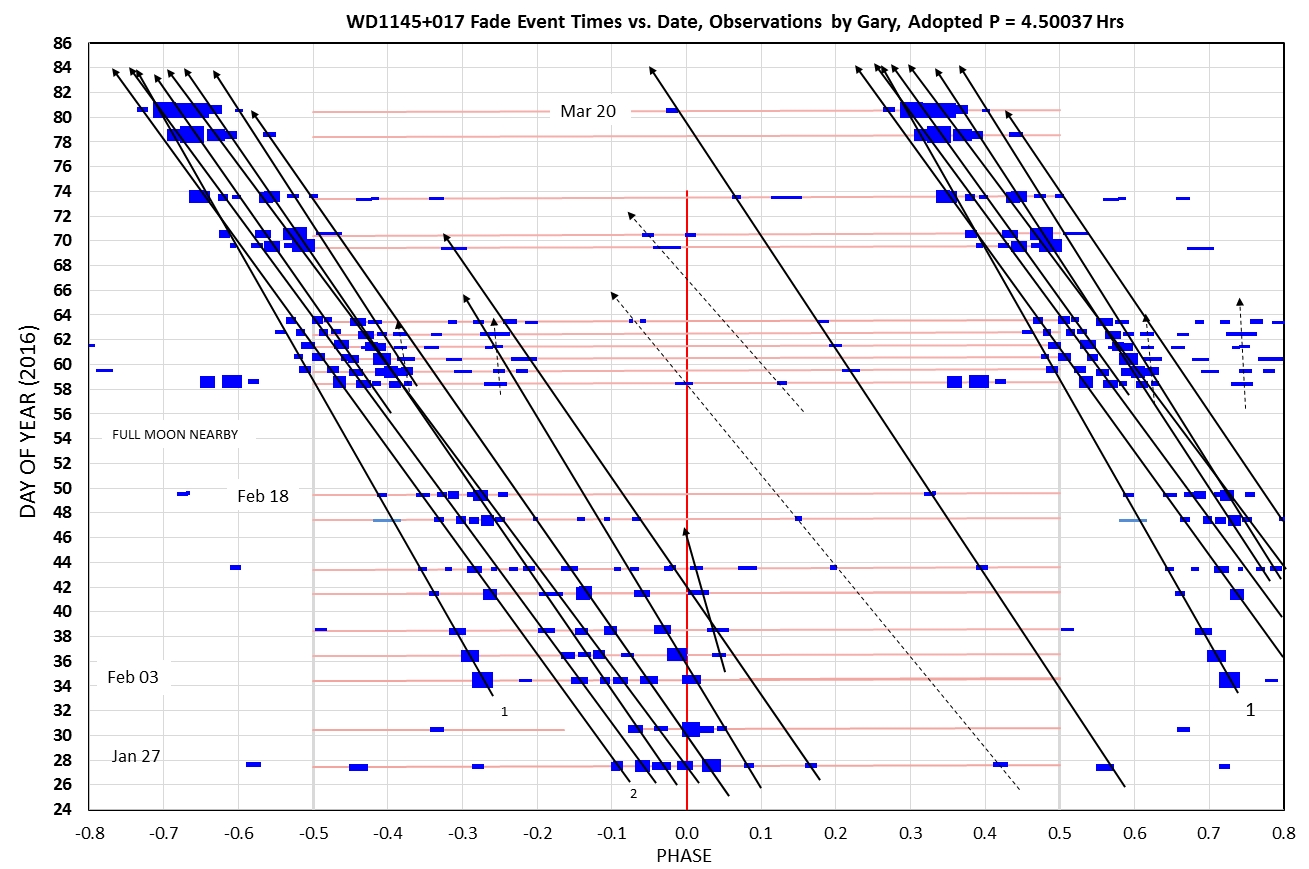
This waterfall plot
is for data taken after the MNRAS publication,
Rappaport et al (2016).
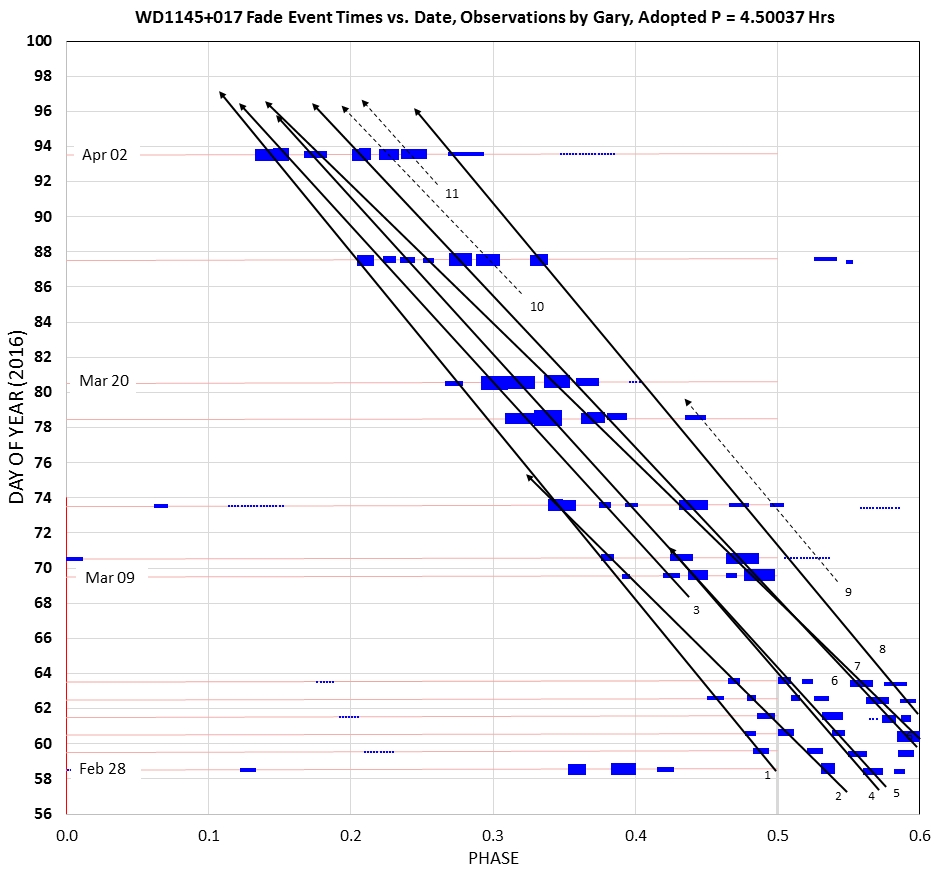
Expansion of
above figure, showing detail of the current dip
group. The average drift rate for this group is
-2.80 min/day.
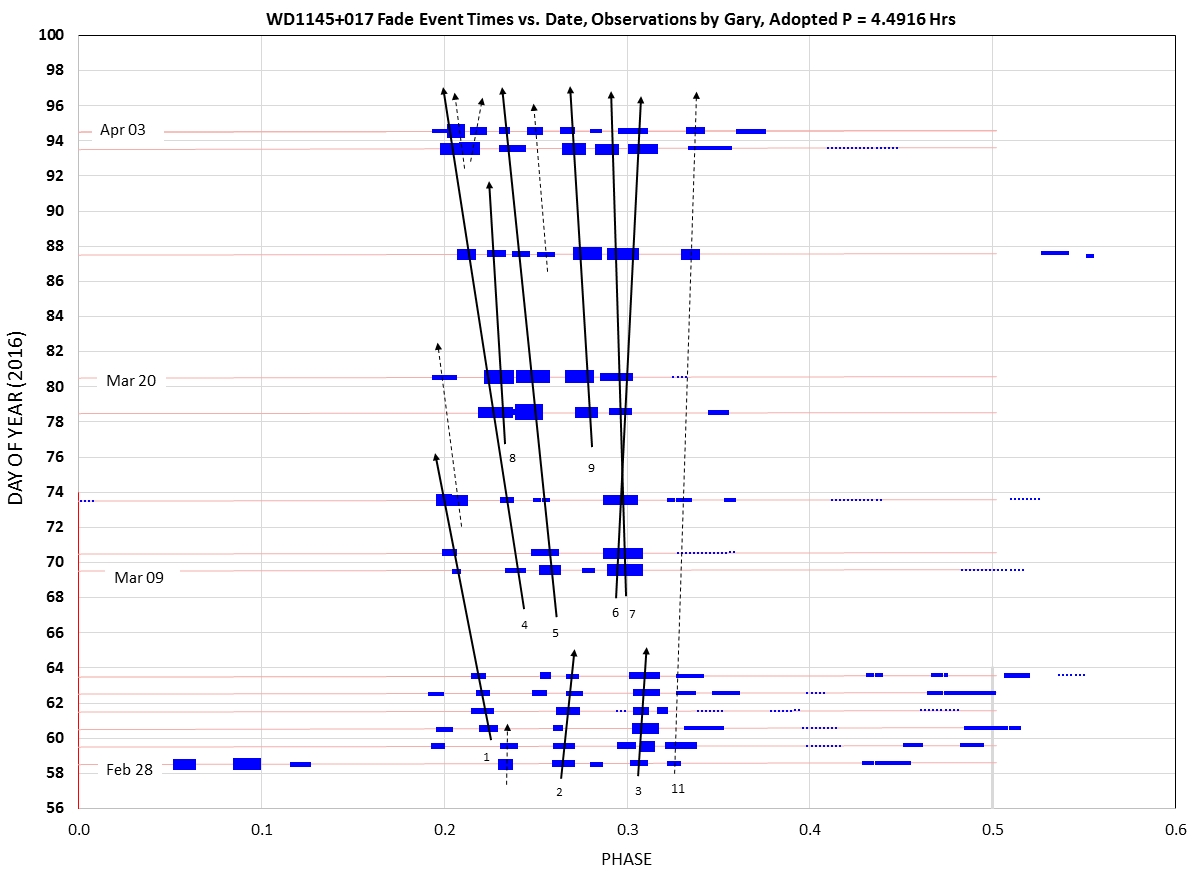
This
waterfall uses the fragment period, so drift lines
are close to vertical (which is easier to
interpret).
The current group of dips drifts with respect to
the A-period (4.50037 hrs) at a rate of -2.80
min/day. The fragments producing dust clouds
therefore have a period of 4.4916 hrs (0.187151
day). The dip structure therefore appears 1.5419
hours earlier each day (i.e., 0.064246 days earlier
each day). An ephemeris for the center of the dip
structure is 2457481.7458 + 0.187151 × E.
There's another series of waterfall plots at
this web page: link
It shows activity level vs date for each drift line
(fragment) for a limited interval of dates in early
2016.
B Orbit Dip Period
So
far only 1 dip has appeared with the K2 B period
of ~ 4.6053 hrs.
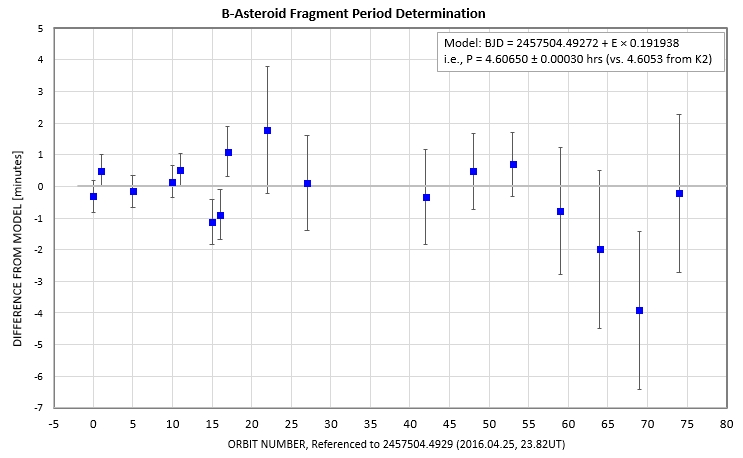
The
fast-moving dip that suddenly appeared on 2016
Apr 26 has a period of 4.6066 ± 0.0004 hours,
which is the same as the K2 B period. It must
therefore be either a fragment that recently
left the B-asteroid, or the B-asteroid is
producing a dust cloud. It has a much longer
trailing tail than leading one.
Spectral Energy
Distribution (SED)
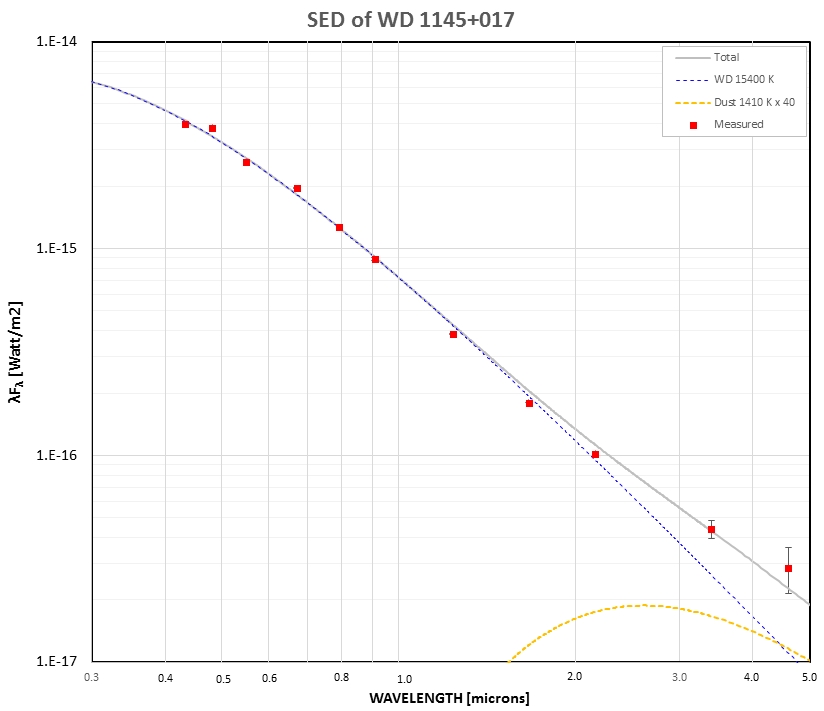
SED based on
measured B-mag & V-mag (using 12 nearby
reference stars). Other mag's can be estimated
using the fitted BB model.
Fragment Periods
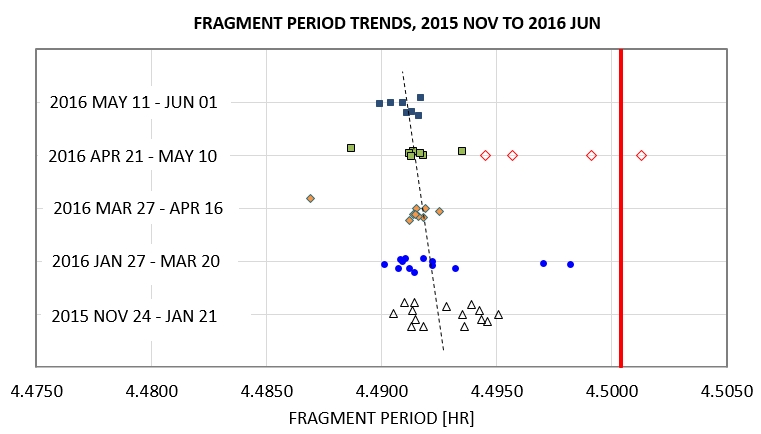
It appears
that the fragment period has been decreasing
during the past 7 months.
The
Poet Within Me
When a neighbor asked
what I’ve been doing lately, the poet within me
spoke up: I’ve been getting a far-future glimpse
of our sun, the Earth and the matter that makes up
you and me. I told how our sun will swell to giant
size, then collapse into a white-hot dwarf star
the size of Earth, followed by surviving planets
disintegrating, breaking off pieces, some of which
will include the atoms that once were you and me,
and that we will in this way contribute to dust
clouds that will obscure some of our diminutive
sun's light, to be noticed by some intelligent
observer elsewhere in the galaxy, curious to
understand what's out there, and perchance to
better know their future. I presume that those
observers will be as clueless about the privileged
time of their race as ours was while living
through the best of times for us during the
mid-20th Century on this wondrous home planet. At
our human high noon, when our fortunate species
prospered and squabbled upon a giving Earth, we
forgot to feel gratitude. Yes, gratitude was the real
subject for what I had been doing lately;
it always comes when the grateful thing is gone,
as it certainly will be when our horrible human
race, with its deep-rooted tribal mentality,
transforms an artisan created civilization into a
cancer that chokes off most life on Mother Earth,
eventually including itself. My neighbor politely
nodded, pretending to understood.
Evolution of the
Culture of Astronomy and the State of Pro/Am
Collaborations
It has taken me about a decade to figure
out that the astronomy culture of today has changed
from what I knew ~ 5 decades ago, when I was a
professional radio astronomer.
During the 1960s and 70s the
culture was collegial; astronomers helped each
other get established, and establishing a career
required only a +2-sigma IQ and a love of the
subject. We helped each other, and shared what
we were doing without suspicion. During the 70s
I transitioned into the atmospheric sciences,
and lost track of the culture of astronomy,
except for occasional grumblings by former
colleagues. I recall MJ complaining, during the
1980s, that getting funded required being part
of an "old boy's club."
After retiring in 1998 I remembered the fun of
my childhood astronomy hobby, before college, so
I bought a 10" Meade telescope and returned to
astronomy as a hobbyist, an amateur. The
transition from film to CCD was occurring, and
as a result new observing opportunities for
amateurs were opening up. I became one of the
first amateurs to measure an exoplanet transit
in 2002. I urged other amateurs to join in this
fun, and created a web page for archiving
amateur exoplanet transit LCs (AXA,
with ~640 LCs). I tutored many of these amateurs
so they could produce scientifically useful
light curves. A few professional astronomers
took note, and I was invited to collaborate by
observing exoplanet candidate stars (the XO
Project). Later I began a consulting arrangement
with Vanderbilt University (i.e., KELT), lasting
~ 3 years.
I slowly started to take a new reading of the
professional astronomy culture. It seemed that
the most striking change was an out-of-balance
supply/demand ratio; in other words, there
were too many young PhD astronomers
competing for the funding
available from NASA and NSF. It occurred to me
that universities were graduating too many PhD
astronomers (because it allowed them to maintain
teaching staff).
After a couple years consulting for an asteroid
astronomer, I gained some insight into the
funding atmosphere for that discipline. That's
where I learned about the new need for sharp
elbows, keeping secrets and bitter competition
for recognition. More recently I learned
something from working with a young WD
astronomer, in which my role was creating a
group of amateurs for providing all the
observations.
I have come to realize the role of secrecy in
creating a career in astronomy. Using others
without their knowing it is also helpful.
Accepting co-authorship without contributing is
acceptable. Anything, in short, that promotes
securing employment defines the new astronomy
culture. I'm not overlooking the role of
intelligence and knowledge; it's just that those
are merely a minimum requirement. Having a
+3-sigma IQ, and being highly motivated, are
just the entry ticket for achieving a career in
astronomy because those other new skills that
I've recently learned about have become
important. I sense the loss of "spontaneous fun"
within this new culture, so "fun" may now be
secondary. And there's also a loss of trust. It
is now necessary to think twice about a
colleague's motives. For example, does that
person have an affiliation with another group of
astronomers doing work that competes with what
we're doing together, and is he passing secrets.
Who is going to beat us in publishing similar
work, knowing from an informant our publishing
schedule.
For some people, this may be fun; but not me!
I'm old fashioned, and accustomed to openness
and trusting relationships. If this is
unfeasible, then count me out. I'll restrict my
future collaborations to other amateurs. For
this reason I am proud to be an amateur again!
The rest of what I once wrote for this section might
be considered a "rant" that's a bit too candid for
this web page. If anyone wants to read it you'll
have to ask me for the web page PW. The username is
"123" but to get the PW you'll have to e-mail me
(see bottom of this page). The rant is located at link.
Another complaint that I've slowly come to
recognize, which I think afflicts the present community
of astronomers, is what I call the "Culture of Caution."
The best way to convey what I'm referring to is with a
joke that Dr. Gulkis told me 5 decades ago, shortly
after I hired him at JPL:
A scientist is traveling
on a train, and a traveling companion remarks "Look at
that brown cow grazing in the field." And the
scientist replies "Well, we only know it's brown on
the side we can see." This joke may only be funny
for someone who has had to work with professional
scientists and felt the frustration of getting past a
reasonable assumption for the sake of pursuing an
interesting speculation. That's the frustration I felt
when Tom Kaye and I tried persuading a professional
astronomer on our WD1145 team that we were seeing dip
features drift to earlier times using the A-asteroid
ephemeris. I think it took 3 or more weeks of data to
sort of convince him, and he's still very cautious about
believing my drift lines. He also seems reluctant to
accept the use of converging drift lines as a way of
assigning a creation date for a fragment collision. Some
favorite paragraphs, and one section, in the
MNRAS
manuscript, were deleted before submission for lack of
supporting theory. When we received a 3-page list of
comments from an anonymous reviewer assigned by the
MNRAS
journal, the same excess of caution was present.
Basically, "Can you prove that the drift lines that you
created by eye are physically associated?" (My response,
kept to myself: "Of course not, but can't we be
reasonable!"). It was like asking if I could prove that
the cow was brown on the other side. Partly in response
to the reviewer comments (urging caution), an additional
section (and my favorite figure) were deleted from the
journal article. I feel like the article has been
eviscerated of much of what made the WD1145 observing
and speculation project fun. From now on, I'm going to
avoid the killjoy "Culture of Caution" by only
"publishing" in web page format, and maybe supplemented
by PDF document summaries (as in
link).
Finder Image
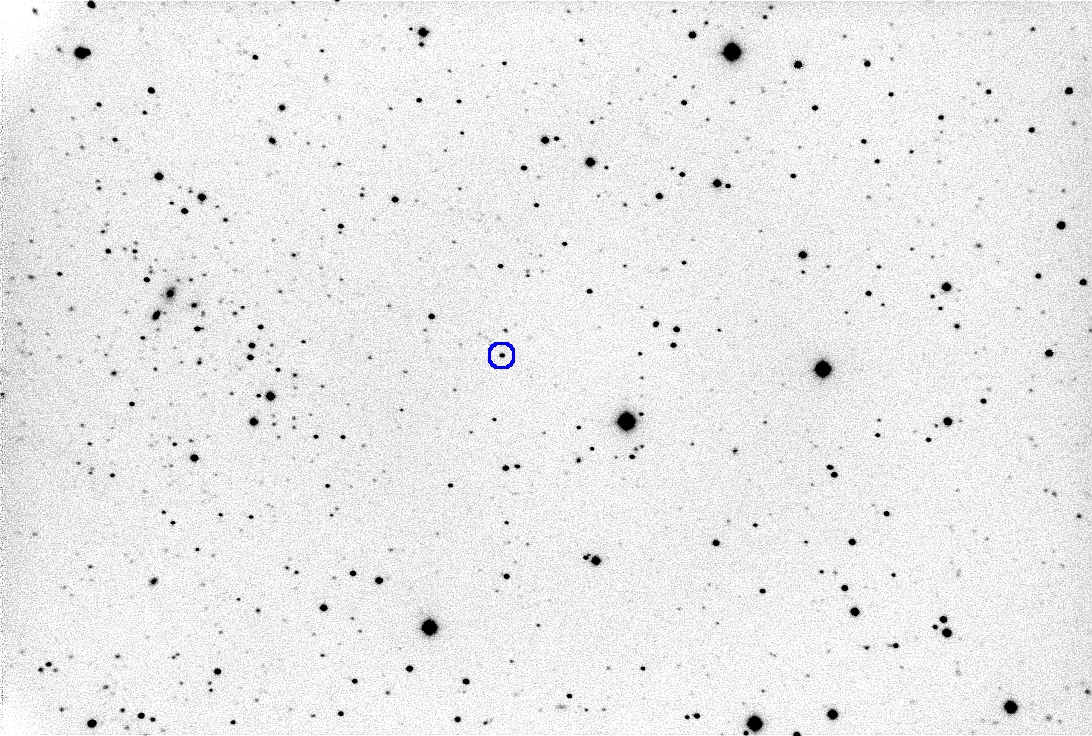
WD1145 is at 11:48:33.59 +01:28:59.3
(J2000). FOV = 27 x 18 'arc, northeast at upper
left.
References
Vanderburg
et al, 2015,
"A Disintegrating Minor Planet Transiting a White
Dwarf,"
Nature, 2015 Oct 22,
arXiv:1510.063387
Croll
et al, 2105,
"Multiwavelength Transit Observations of the Candidate
Disintegrating Planetesimal Orbiting WD 1145+017,"
ApJ,
arXiv:1510.06434
Gaensicke et al, 2015, "High-Speed
Photometry of the Disintegrating Planetesimal at WD
1145+017: Evidence for Rapid Dynamical Evolution,"
arXiv
:1512.09150
Gary, B. L., S. Rappaport, T. G.
Kaye, R. Alonso and F.-J. Hambsch, "WD 1145+017
Photometric Observations During 8 Months of High
Activity," 2017, MNRAS,
465,3267-3280.
Abstract
&
PDF,
arXiv:1608:00026
Rappaport, S., B. L. Gary, T.
Kaye, A. Vanderburg, B. Croll, P. Benni and J. Foote,
2016, "Drifting Asteroid Fragments Around WD 1145+017,"
MNRAS,
arXiv:1602.00740
Alonso, R., S. Rappaport, H. J. Deeg
and E. Palle, 2016, "Gray Transits of WD 1145+017 Over
the Visible Band,"
Astron. & Astrophys.,
arXiv:1603.08823
Petit, J.-M and M. Henon, 1986,
Icarus,
66, 536-555 (
link)
Redfield, Seth, Jay Farihi, P. Wilson,
Steven G. Parsons, boris T. Gansicke and Girish Duvvuri,
"Spectrosopic Evolution of Disintegrating Planetesimals:
Minutes to Months Variability in the Circumstellar Gas
Associated with WD 1145+017,"
arXiv:1608.00549
Xu, S., M. Jura, P. Dufour & B.
Zuckerman, 2016,
ApJ,
816, L22
Veras, Dimitri, Philip J. Carter, Zoe
M. Leinhardt and Boris T. Gansicke, 2016,
arXiv
Tom Kaye presentation at 2016
Society for Astronomical Science meeting:
link
Related Links
Mukremin Kilic's pro/am monitoring
of dusty WDs: link
Tom Kaye video of talk about WD1145
presented at Society for Astronomical Sciences(SAS),
June, 2016: link
Some observing "good practices" for
amateurs (book): Exoplanet
Observing for Amateurs
Hereford Arizona Observatory
(HAO): http://www.brucegary.net/HAO/
Tutorial for faint object observing
techniques using amateur hardware: http://brucegary.net/asteroids/
Master list
of my web pages & my Resume